Following the new conceptual framework outlined on page 3, these Research highlights are identified as contributing to understanding Earth Architecture (the roadmap for fluids) and/or Fluid Fluxes (the “traffic report”), with logos for easy attribution. For a full description of the foundation projects, see Appendix 1.
Deep Earth recycling in the Hadean
Could the mixing of Earth’s earliest geochemical reservoirs constrain the geodynamics of the Earth during the Hadean period?
The Hadean has been referred to as a geological dark age – for the first 500 million years of Earth’s history, there is a complete dearth of any geological record. The crust of this time was probably destroyed by meteorite bombardment, volcanic resurfacing, vigorous tectonic mixing or all three.
The Hadean was undoubtedly hellish – on top of the heat supplied by relentless meteorite bombardment, the mantle was efficiently heated by high rates of radioactive decay, including short-lived, and now extinct, isotopes like aluminium-26. The mantle also retained much of the substantial heat generated during accretion and the formation of the core.
Under this sort of hot mantle conditions, traditional wisdom has it that the interior viscosity of the Earth would be quite low, and mantle convection extremely vigorous. Mantle convection simulations have suggested that the mixing of early geochemical reservoirs should have been extremely efficient under these conditions, and should have been homogenised on timescales of less than 100 Myrs.
Recently, a number of lines of evidence have provided contrasting views on the Hadean. Model ages on the Nuvvuagittuq greenstone in Quebec suggest some Hadean crust has survived, and zircons from Jack Hills have been used to argue for subduction processes in the Hadean. However, the residence time for the mafic protolith for these Jack Hills zircons is ~400 Myrs – an inordinately long time for a thick mafic crust to survive on an active mantle.
The preservation of geochemical anomalies in younger Archaean rocks also suggests long survival times and inefficient mixing in the Hadean. Neodymium-142 anomalies reflect very early crustal extraction – and the identification of such anomalies in 2.666 Ga basalts from the Abitibi belt suggest these mantle anomalies survived for almost ~2 Gyr. Similarly, tungsten-182 anomalies reflect primordial partitioning events, yet are still observed in rocks as young as 2.8 Gyr from the Kostomuksha Greenstone belt in the Baltic Shield, Russia. Lastly, the present concentration of platinum-group elements in the Earth’s mantle is thought to be due to a post-core meteoritic addition, called the late veneer. However, PGEs did not reach their present concentration in mafic lavas until ~2.9 Ga; prior to that they increase linearly from about 3.9 Ga onwards – suggesting they were being progressively mixed into the deep mantle and sampled during this time.
The mixing times of these independent geochemical reservoirs (~2 Gyr) are far longer than the anticipated mixing time from simple convection simulations (<~100 Myr), and so the question becomes – what is missing?
The answer may lie in the tectonic response of the Earth to its hot, early start. New work at CCFS by Craig O’Neill and colleagues suggests that for most of the Hadean the Earth may have been tectonically inactive – the low interior viscosities prevented the build-up of the stress required to fracture and mobilise plates, and the planet may have been in a “hot stagnant lid” regime. The evolution from this starting point into a tectonically episodic regime with rapid, recurring overturn events, has profound implications for Earth’s mixing history and thermal evolution.
Three dimensional spherical-cap simulations (pictured), starting from a hot stagnant-lid state, suggest the mixing time in such a regime is an order of magnitude less than for either plate tectonics, or the simple convection calculations performed previously. This in itself can explain the discrepancy between observed mixing times, and those predicted by earlier models.
On top of that, the new simulations suggest that the early Earth lost its heat inefficiently. Previous thermal-evolution models for Earth invariably run into a problem called the “Archean thermal catastrophe” – where in order to match declining heat fluxes, internal temperatures would have been unreasonably high (i.e. global mantle melting) during the Archean. The new models prevent this thermal catastrophe, by demonstrating that Earth could have lost its heat inefficiently, and had lower global heat fluxes early in its history than previously assumed - meaning that internal temperatures could stay within reasonable bounds.
This project is part of CCFS Theme 1, Early Earth, and contributes to understanding Earth’s Architecture and Fluid Fluxes.
Contact: Craig O’Neill
Funded by: ARC Future Fellowship; MQ infrastructure funding
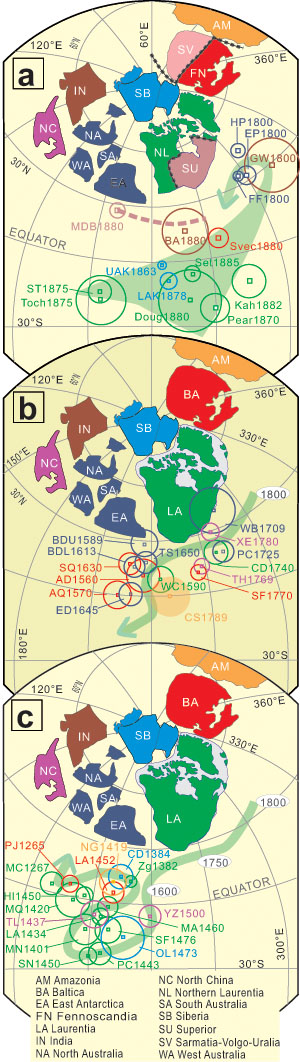
Synchronous pole dancing: the state of supercontinent Nuna before Rodinia
The idea that Earth’s evolution has been dominated by cycles of supercontinent assembly and breakup has been debated for decades. However, our understanding of past supercontinents has mainly been limited to the last 1000 Ma; we could be more confident about the history of the ca 320-180 Ma supercontinent Pangea than the ca 900-700 Ma supercontinent Rodinia. Our knowledge of an even older supercontinent (Nuna or Columbia), formed around 1.8 Ga, has been more tenuous, and early papers were mostly based on intercontinental geological correlations that are intrinsically non-unique. Early attempts at Nuna-related continental reconstructions were hampered by the lack of high-quality palaeomagnetic results, or merely regional, rather than global considerations.
Recently (CCFS publication# 197) we reported new palaeomagnetic results from North China, and by integrating recent results from Australia, India, and Amazonia and all previously reported results, we reconstructed the first near-complete reconstruction of Nuna (Fig. 1). Our work indicates that Nuna formed by ca 1750 Ma, and lasted at least until ca 1400 Ma (Fig. 1 and 2). This work was involved an ongoing international collaboration with Professor Shihong Zhang of China University of Geosciences, and Professor David Evans of Yale University.
Figure 1. Presently available paleomagnetic datasets enabled the reconstruction of the first palaeomagnetically constrained complete Nuna supercontinent (Zhang et al., 2012; CCFS contribution #197), all in present North American coordinates. Cratons and their poles are colour coded, and ages following pole abbreviations are in millions of years before present (Ma). (a) Palaeomagnetic poles between 1880 and 1800 Ma, showing broad convergence (light-green swath) of poles from the Slave craton of Laurentia, Siberia, Baltica, India and Australia, which suggests that these blocks might commence to join together at ca 1800 Ma. Arrow points toward the position of younger poles. (b) Poles between 1780 Ma and 1600 Ma from Australian cratons, Laurentia, Baltica and NCB, define a common apparent polar wander path (APWP) for Nuna (green curve); arrow points toward the position of younger poles. (c) Palaeomagnetic poles for 1500 -1265 Ma from North China, Siberia, Laurentia, Baltica and Amazonia against the common APWP (green curve) that is defined by all poles between 1800 Ma and 1260 Ma, indicating common motion to at least 1380 Ma. Arrow points toward the position of younger (< 1200 Ma) poles from Laurentia and Baltica. See CCFS contribution 197 for more details.
Our reconstruction agrees with previously proposed, geologically based models, including the SAMBA connection between Baltica, Amazonia and Western Africa, the Nuna core connection between Laurentia, Baltica and Siberia, the proto-SWEAT connection between Laurentia, Mawson block and Australian blocks, and the North China-India connection. In addition, our reconstruction for the first time quantitatively merges these regional connections into a single and coherent supercontinent.
Our Nuna reconstruction, constrained by both our new results and an updated global paleomagnetic dataset, is also consistent with key geological features including the ca 1800 Ma orogenic belts leading to the assembly of Nuna (Fig. 2a) and Mesoproterozoic intraplate extensional basins and the Large Igneous Province (LIP) record that may be related to the breakup of Nuna (Fig. 2b). This breakup may have begun around 1400 Ma ago, but available paleomagnetic data are not yet complete enough to allow a more precise depiction of Nuna’s fragmentation.
This research is directly related to CCFS foundation project 6: Detecting Earth’s rhythms, and projects 2d and 9.
This project is part of CCFS Theme 2, Earth Evolution, and contributes to understanding Earth’s Architecture.
Contacts: Zheng-Xiang Li, Shihong Zhang, and David A.D. Evans
Funded by: Chinese National Natural Science Foundation grants and 973 Program support, ARC discovery grant (DP0770228), and CCFS Foundation Project 6
Figure 2. Configuration and palaeogeographic position of the supercontinent Nuna (a) at ca. 1740 Ma soon after its assembly, and (b) at ca. 1590 Ma, when large igneous provinces and extensional basins were extensively developed. Paleomagnetic poles from Laurentia were used to plot the paleolatitude of the supercontinent. Placing Laurentia in northern hemisphere was based on the likely westward trade-wind direction interpreted by Hoffman and Grotzinger (1993) from the 1950 -1860 Ma geological records in the Slave craton. We turned the world upside-down to make Australia and North America plotted upright, and therefore for easier viewing. See CCFS publication #197 for more details.
More insights from Earth’s oldest zircons: ~4.45 Ga crust in the North Qinling Orogenic Belt, China
Most of what we know about Earth’s earliest crust comes from Jack Hills in Western Australia, where hafnium-isotope data from old detrital zircons in a younger sandstone indicate that their parent magmas were melted from still older rocks that formed about 4.4-4.5 billion years (Ga) ago. These zircons provide our only means of exploring the Hadean period, the time between the formation of the Earth and the preservation of the oldest known rocks, formed about 3.8 Ga ago. However, Hadean zircons have been found in only four other localities; the Southern Cross belt in Western Australia, the Acasta gneiss complex in northwestern Canada, and China. The Chinese examples include a single 4.1 Ga grain from the Burang area of Tibet and another ~4.1 Ga grain from the North Qinling Orogenic Belt. The latter discovery comes from rocks in a Phanerozoic collisional belt developed between the North and South China cratons. The zircon was found in Ordovician volcanic rocks of the Caotangou Group, dated at 456 ± 2 Ma. These arc volcanic rocks penetrated the basement of the North China Craton, so the zircon xenocryst could have been picked up by the magma either in its source region or during its ascent; it is the first report of Hadean crustal material in a Phanerozoic igneous rock.
For this study, over 3000 zircon grains were separated from ignimbrite collected from the same outcrop as the initial discovery, and were analysed for U-Pb age using both SHRIMP and LA-ICP-MS techniques. In total, only three grains were identified with ages ≥3.9 Ga and these were selected for U-Pb and Hf-O isotopic analysis.
The magmatic cores in these zircons range in age from 3909 ± 45 Ma to 4080 ± 9 Ma and record Hf crustal model ages up to 4.5 Ga. Importantly, the latter lie on the same Lu/Hf trajectory as the least-disturbed Jack Hills zircons, so they are only the second example of the earliest known crustal rocks on Earth. The zircon cores also show a wide range in δ18O, both above and below the mantle value (Fig. 1); this is strong evidence that the source rocks of the magmas had been subjected to surficial processes such as weathering. The rims of two grains record new zircon growth at 3.7 Ga and, when combined with their presence in an Ordovician volcanic rock, suggest that ancient crust was still present in the basement of the North China Craton during the Paleozoic (CCFS publication #301 ).
Figure 1. Plot of δ18O versus age for the xenocrystic zircons (yellow symbols) from the North Qinling Orogenic Belt, China. In green is the band for ‘mantle zircon’ (Valley et al., Contrib. Mineral. Petrol., 1998); the orange area shows the field of global >3.5 Ga zircons (from Valley et al., Contrib. Mineral. Petrol., 2005; Harrison et al., Science, 2005; Trail et al., Geochem. Geophys. Geosystems, 2007; Heiss et al., Geochimica et Cosmochimica Acta, 2007).
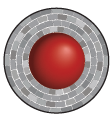
This project is part of CCFS Theme 1, Early Earth, and contributes to understanding Earth’s Architecture.
Contact: Simon Wilde
Funded by: CCFS Foundation Project 2; The National Basic Research Program of China (973 Program; grant No. 2012CB416601), the National Natural Science Foundation of China (NSFC; grant No. 41272004) and the MOST Special Funds from the State Key Laboratory of Continental Dynamics, Xi’an, China
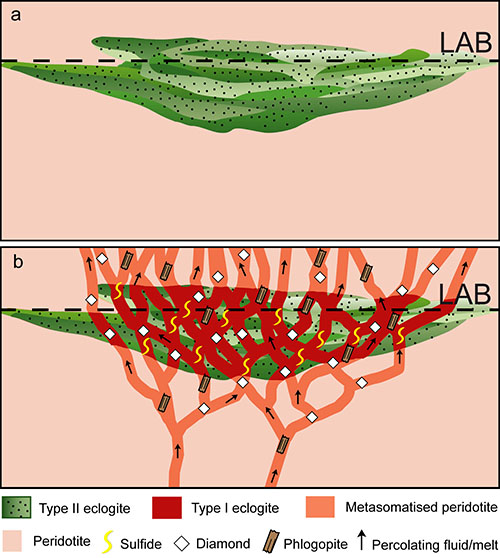
Unmasking xenolithic eclogites: sleuthing with the key samples
Xenolithic eclogites are high-temperature and high-pressure mafic rocks, generally brought up from 150-220 km depth to Earth’s surface by kimberlites. They are minor but important constituents of the subcontinental lithospheric mantle (SCLM), and information on their evolution and origin can provide unique constraints on the history of the ancient lithospheric mantle.
Extensive studies of eclogite samples from all over the world have generated two contradictory hypotheses about their origin. One regards the eclogites as deep-seated magmatic rocks, while the other regards them as components of subducted oceanic slabs. To test these hypotheses, it is essential to find out whether the samples being studied actually contain the information we are after (i.e. what is the RIGHT sample).
Previous work on the famous eclogite suite from the Roberts Victor kimberlite (South Africa) has divided the samples into Types I and II. Type I eclogites are heavily metasomatised by a sequence of melts/fluids in the carbonatitic-kimberlitic spectrum; Type II eclogites may be the protoliths of Type I (Fig. 1; CCFS publications #3, 41).
Figure 1. Cartoon of the Roberts Victor eclogite body at the base of SCLM before (a) and after (b) the metasomatism.
The progressive metasomatism inferred from studies of the whole eclogite suite has now been found within one hand specimen, RV07-17 (Fig. 2). From top to bottom, this sample becomes less equilibrated in terms of microstructure; grain boundaries that are smooth in the upper part become convolute and complex in the lower part, which also has more secondary minerals (e.g. phlogopite, sulfide) and more fluid inclusions.
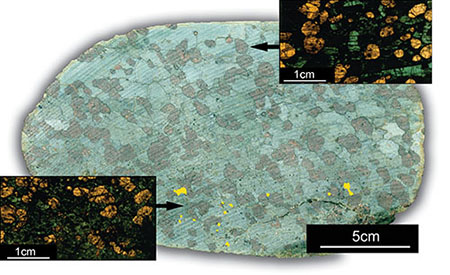
Figure 2. Handspecimen photo of RV07-17 and thin-section images of upper and lower parts of the sample.
Four zones (Zone 1, 2, 3, and 4) can be recognised using the chemical compositions of garnets (Fig. 3). From Zone 1 to Zone 4, the pyrope content of the garnets increases gradually from 0.47-0.62; cpx shows progressive enrichment in MgO (10.8-14%). The cross-cutting pattern strongly suggests that Zone 1 represents an early stage of the metasomatism, and Zone 4 the latest stage.
REE patterns of both garnet and cpx also show systematic changes (Fig. 4a-4b). The garnets of Zone 1 have flat REE patterns from Lu to Sm, but a strong depletion in the LREE. Toward Zone 4, the relative abundance of the MREE of the garnets drops significantly, giving smoother patterns. The large cpx grain in Zone 1 shows a strong depletion in the LREE, but the LREE/MREE of the cpx increases from Zone 1 to Zone 4. The Sr contents of cpx change sharply from ~230 ppm in Zone 1+2 to ~320 ppm in Zone 4, and the 87Sr/86Sr of cpx increases from ~0.7055 in Zone 1+2 to ~0.7063 in Zone 4. From Zone 1 to 4, δ18O of the garnet decreases from ~8.5 ‰ to ~6.0 ‰ as the MgO content increases (Fig. 4c).
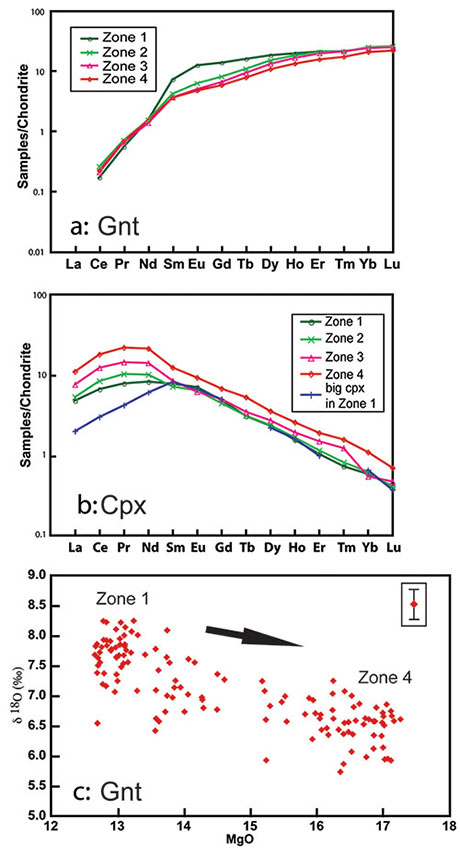
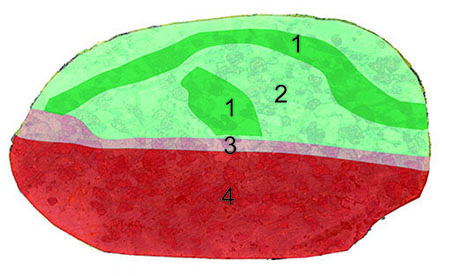
Figure 3. Drawing of RV07-17 showing four different zones.
These observations suggest that the eclogite was metasomatised by Mg-rich melts/fluids, which changed through time from carbonatitic in Zone 1 to more kimberlite-like in Zone 4. This is consistent with the decrease of δ18O in the metasomatic agent from the early carbonatitic fluids to the late kimberlitic melt/fluid.
The metasomatism of some mantle eclogites (e.g. Type I) may be more intensive and complete than previously thought. This process can sweep away all original information on chemical compositions, making it nearly impossible to define a protolith. To know the origin of the xenolithic eclogites, and to use them as evidence for different dynamic scenarios, the least-metasomatised samples must be studied.
This project is part of CCFS Theme 2, Earth Evolution, and contributes to understanding Earth’s Fluid Fluxes.
Contacts: Jin-Xiang Huang, Yoann Gréau, Bill Griffin, Sue O’Reilly
Funded by: ARC Discovery (O’Reilly and Griffin) then CCFS Foundation Project 8, MQRES, EPS Postgraduate Fund
Figure 4. (a) REE patterns of average gnt composition in each of the four zones; (b) REE patterns of average cpx compositions in each of the four zones; (c) correlation between δ18O and MgO of the garnets in the zones.
Rare rocks – the smoking gun for lithosphere drop-off 435 million years ago
How can an orogen (mountain belt) form within a continental plate? Why do these episodes of crustal deformation end with widespread intraplate magmatism? These types of geological activity represent a “hole” in the Plate Tectonics paradigm, which emphasises activity along plate boundaries. The 2000 km-long intraplate Wuyi-Yunkai orogen, active in the early Palaeozoic South China, is well documented. It has high-grade metamorphic rocks in the orogenic core (Fig. 1a, dark grey area), and widespread, mostly late- to post-orogenic granites, in both the orogenic core and the foreland areas (light grey areas; Fig. 1a). Previous regional tectono-magmatic analyses suggested that this widespread late- to post-orogenic granitic magmatism mostly occurred during orogenic collapse when the deep crust (the “root”) of the orogen delaminated. However, the lack of any syn- to late-orogenic mafic rocks made it difficult to verify this or any other possible models.
Figure 1. (a) Simplified regional geological map highlighting the regional extent of the early Paleozoic Wuyi-Yunkai orogeny in South China, featuring high-grade metamorphic rocks (shown in green) in the orogenic core (shown in dark grey), and widespread late- to post-orogenic granites (shown in red) throughout the orogeny (after Li et al., GSA Bull. 2010). (b) Plot of La/Nb versus εNd(435 Ma) for the rare Chayuanshan basaltic rocks in northern Guangdong province, which point to a sub-continental lithospheric mantle (SCLM) source region.
The discovery of rare Silurian post-kinematic basaltic to andesitic volcanic rocks in northern Guangdong province (CCFS publication #193) has made it possible to study mantle-crust interactions during the intraplate orogenic event. The Chayuanshan volcanic rocks crop out on the northwestern margin of the orogenic core (Fig. 1a, marked as “Study area”). They unconformably overlie strongly-deformed Cambro-Ordovician strata, but are in low-angle unconformable contact with overlying post-orogenic mid-Devonian strata. LA-ICP-MS and SHRIMP U-Pb dating of zircons from two andesitic samples gave consistent magmatic ages of 434 ± 6 Ma and 435 ± 6 Ma, which are younger than the 460-440 Ma peak metamorphism of the orogeny but synchronous with the widespread un-deformed, late-orogenic (440-415 Ma) granitic intrusions. Nine basaltic samples, with the least crustal contamination, have high MgO contents (12.3-19.2 wt.%). The Nd isotope and trace-element compositions of these high-Mg basalts point to a source region of an ancient sub-continental lithospheric mantle (SCLM; Fig. 1b), which is generally depleted in basaltic-melt components in comparison with the asthenospheric mantle, resulting in high mantle Mg# values. The calculated primary basaltic melt has ~50 wt% SiO2, ~14 wt% MgO and ~9 wt% FeOT. The estimated potential temperature for the melts is close to 1400 ºC, much higher than that of a normal sub-continental lithosphere. The effective melting pressure for the melts is 1.0-1.4 GPa. This suggests that the magma was generated from partial melting of lithospheric peridotite heated by hot upwelling asthenosphere. The related high-Mg# andesites are interpreted as the products of differentiation and assimilation, fractionation and contamination processes from the same basaltic magma source, as supported by their negative zircon εHfT values (-21.7 to -6.3) and high zircon δ18O values (7.3-9.0 permil).
Our results lend strong petrological support for the idea of an orogenic root delamination beneath the Wuyi-Yunkai orogeny. As shown in Figure 2, we interpret the Chayuanshan basaltic rocks as representing partial melts near the edge of the remaining sub-continental lithospheric mantle (SCLM). Heat from the upwelling asthenosphere as well as possible basaltic underplating, and regional decompression caused by orogenic collapse, can best explain the widespread synchronous granites along the orogen. In this model, the low- pressure and high-temperature melting of a hydrated subcontinental lithospheric mantle, as demanded by the basaltic magmas, can be satisfied by the delamination of the root, and the consequent orogenic collapse. The volcanic rocks unconformably overlie the strongly deformed Cambro-Ordovician succession, but are nearly parallel with the overlying, post-orogenic Devonian succession; this is consistent with a late-orogenic origin. The ca 435 Ma age of the volcanism post-dates the high-grade metamorphism at ca 460-440 Ma, but is synchronous with the widespread, dominantly post-kinematic felsic magmatism at ca 440-415 Ma. The underplating of mafic magmas, in combination with heat from the upwelling asthenosphere and decompression due to orogenic collapse, provides a plausible explanation for the widespread post-kinematic felsic magmatism.
Figure 2. A cartoon diagram showing the delamination of the sub-continental lithospheric mantle (SCLM) and lower crust after crustal thickening during the intraplate Wuyi-Yunkai orogeny at ca 435 Ma.
This project is part of CCFS Themes 2 and 3, Earth Evolution and Earth Today, and contributes to understanding Earth’s Architecture and Fluid Fluxes.
Contacts: Wei-Hua Yao, Zheng-Xiang Li, Xuan-Ce Wang, Xian-Hua Li
Funded by: Chinese Academy of SciencesAS SAFEA(KZCX2-YW-Q04-06), NNSFC (40973025) and ARC (DP110104799)
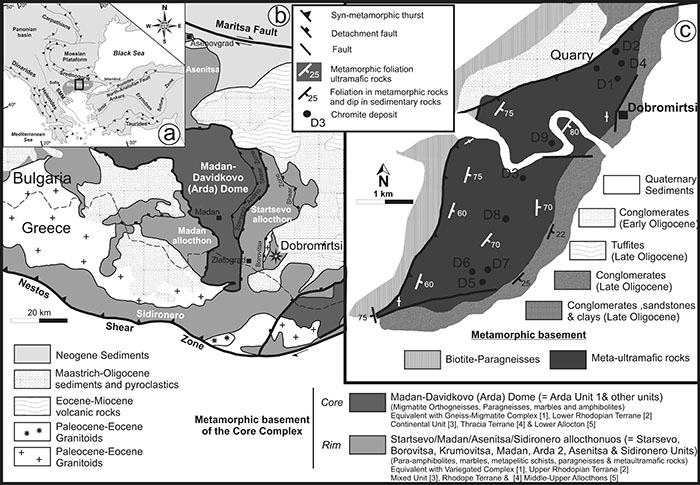
Mobility of osmium unearthed: unexpected consequences for tracking mantle evolution
The Os-isotope compositions of platinum-group minerals (PGMs) in ophiolite chromitites are commonly regarded as resistant to fluid-related processes, and have been used to track the evolution of Earth’s convecting mantle. However, we have found significant differences in 187Os/188Os between primary and secondary PGMs from metamorphosed ophiolite chromitites of the Dobromirtsi Ultramafic Massif, in the Central Rhodope Metamorphic Core Complex of southeastern Bulgaria (CCFS publication #42). Primary (magmatic) PGMs hosted in unaltered chromite cores have 187Os/188Os from 0.1231 to 0.1270, and 187Re/188Os ≤ 0.002. TMA and TRD model ages, calculated relative to the Enstatite Chondrite Reservoir, cluster around three main peaks: ca. 0.3, 0.4, and 0.6 Ga. Secondary PGMs, produced by alteration of magmatic PGMs, have a wider range of variation (187Os/188Os = 0.1124–0.1398, 187Re/188Os ≤ 0.024); these grains yield TMA and TRD model ages from -1.7 Ga up to 2.2 Ga. The larger range in 187Os/188Os in the secondary PGMs is interpreted as due to reactions between the primary PGMs and infiltrating metamorphic-hydrothermal fluids with a range of Os-isotope compositions. This redistribution of Os in PGMs during metamorphism has significant implications for the interpretation of both whole-rock and in-situ Os-isotopes.
Figure 1. Location of the Dobromirtsi ultramafic massif in the Central Rhodope Core Complex in SE Bulgaria
The fact that secondary PGMs in the metamorphosed chromitites of Dobromirtsi yield 187Os/188Os within the range of depleted to enriched mantle sources suggests that much of the Os-isotopic variability previously reported for PGMs taken out of their microstructural setting (e.g. mineral concentrates or detrital grains collected from streams), and interpreted as a magmatic feature, may instead be related to secondary alteration processes. Therefore, interpretations of mantle events based on the in-situ analysis of PGM nuggets from placers may need to be reconsidered. On a more positive note, the Os-isotope data from the secondary Os-bearing phases in ophiolites can actually give a wider perspective on the sources and evolution of the host mantle peridotite.
This project is part of CCFS Themes 2 and 3, Earth Evolution and Earth Today, and contributes to understanding Earth’s Architecture and Fluid Fluxes.
Contacts: José María, González-Jiminéz, Bill Griffin, Norman Pearson, Sue O’Reilly
Funded by: ARC CoE CCFS Foundation Project 1 (TARDIS-E); ARC Post-Award fund to CCFS; grants to international collaborators
Figure 2. a. reflected-light image of massive chromitite. b. BSE image of zoned chromite; unaltered core mantled by secondary chromite enriched in Cr and Fe2+ and depleted in Mg and Al. c. Zoned chromite (BSE); modified core with two secondary rims: an inner rim of Cr- and Fe2+-rich and Mg- and Al-depleted chromite, and an outer rim of Fe3+- rich (ferrian) chromite. d. Homogenous grain (BSE) of secondary ferrian chromite.
Solving the biggest jigsaw puzzle in Solid-Earth Sciences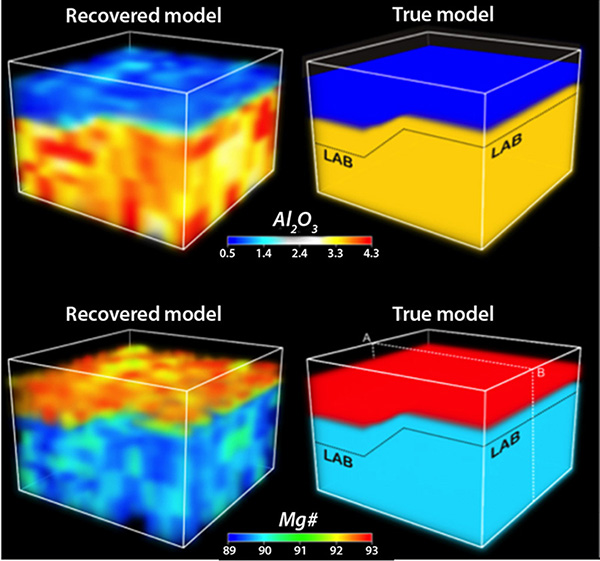
The conversion of geophysical data (e.g. seismic travel-time curves, gravity anomalies, surface heat flow, etc) into robust estimates of the true thermochemical structure of the Earth’s interior is one of the most fundamental goals of the Geosciences. It is the physical state of the deep rocks that drives processes such as volcanism, seismic activity and tectonism. Detailed knowledge of the thermal and compositional structure of the upper mantle is an essential requirement for understanding the formation, deformation and destruction of continents, the physical and chemical interactions between the lithosphere and the convecting sublithospheric mantle, the long-term stability of ancient lithosphere, and the development and evolution of surface topography.
Figure 1. Left: “True” and recovered solution from a full 3D inversion, including travel time residual tomography, using the new method/code for the compositional field. Note that although the absolute magnitudes are not well recovered, the general trend and geometries are correctly identified.
Our current knowledge of the thermal and compositional structure of the lithosphere and the sublithospheric mantle essentially derives from four independent sources.
(i) The most widely applied modelling approach uses gravity and/or surface heat flow data to obtain a model for the temperature and/or density structure of the lithosphere that fits the data to some acceptable level.
(ii) The second most common approach applied to the lithosphere and upper mantle is based on the modelling of seismic data. The aim here is to test thermal and mineralogical (or density) models that are compatible with seismic data (usually shear waves) by using either thermodynamic concepts and/or experimental data from mineral physics. Typically, these studies do not invert directly for composition but rather assume a priori “representative” compositional models.
(iii) A third source of independent information is provided by models and data derived from magnetotellurics (MT). MT is a natural-source electromagnetic method based on the relationship between the temporal variations of electric and magnetic fields at the Earth’s surface and its subsurface electrical structure.
(iv) Finally, the only direct approach is the petrological-geochemical estimation of thermobarometric and chemical data from xenoliths (fragments of upper mantle brought up to the surface by volcanism) and exhumed mantle sections. Where specific mineral assemblages are present, xenoliths can be used to derive the compositional and paleo-thermal structure beneath specific localities.
At present, there are often significant discrepancies between the predictions from these four approaches. The key to progress lies in integrating data from all of these sources into a single consistent model.
Figure 2. Top: Vertical slice from the 3D synthetic model (left) showing the Vp anomaly structure of the true model. Bottom: Recovered solution; ray traces used in the inversion are shown (modified from results presented at the AGU Fall meeting, 2012).
We have developed the first nonlinear, 3D, multi-observable inversion method, based on a probabilistic (Bayesian) inference approach, that can simultaneously invert Rayleigh and Love (seismic waves) dispersion curves, body-wave seismic tomography, and magnetotelluric, geothermal, petrological, gravity, elevation, and geoid datasets. Assembling this giant “jigsaw puzzle” problem has required a massive collaborative effort between thermodynamicists, geophysicists and geochemists, and is the first step towards real thermochemical tomography of the Earth, which is undoubtedly the future of imaging techniques. Our preliminary results (recently published in Journal of Geophysical Research, online Feb 2013) indicate that we can expect to resolve temperature anomalies of ΔT > 150 °C and large anomalies of ΔMg# > 3 (or bulk ΔAl2O3 > 1.5) simultaneously when combining high-quality geophysical data. This resolving power is sufficient to explore some long-standing problems regarding the nature and evolution of the lithosphere (e.g. vertical stratification of cratonic mantle, compositional vs temperature signatures in seismic velocities, etc.) and offers new opportunities for joint studies of the structure of the upper mantle with unprecedented resolution.
This project is part of CCFS Theme 3, Earth Today, and contributes to understanding Earth’s Architecture.
Contacts: Juan Carlos Afonso, Yingjie Yang
Funded by: Discovery Project DP120102372, MQ iPRS
Zircon multi-isotopic mapping: a robust roadmap to mineral discovery
Recent studies in the Yilgarn Craton of Western Australia have demonstrated that multi-isotopic maps, based on in-situ U-Pb and Lu-Hf analyses of zircon and whole-rock Sm-Nd data, are a powerful tool for mapping crustal growth, and for imaging lithospheric blocks and their margins.
These studies pointed to a strong spatial correlation between lithospheric boundaries and the location of large concentrations of several styles of mineral deposit (e.g. Champion and Cassidy, 2007; McCuaig et al., 2010; Begg et al., 2010; Mole et al., 2012). The implication is that these isotopic boundaries mark lithosphere-scale structures that control fluid flux, and thus the location of large mineral systems through time. However, the only available case study in the Archaean is the Yilgarn of WA, and even that is only focused on the central portion of the craton. Therefore, it is critical to test this hypothesis in other parts of the world.
Figure 1. Simplified geological map of Wabigoon Subprovince in western Superior Craton, Canada. The inset shows the location of the study area. The subprovince has been divided into the Winnipeg River, Marmion, Western Wabigoon, and Eastern Wabigoon terranes based on whole-rock Nd-isotope data. The locations of gold and copper mineralisation are highlighted to show their relationship with terrane boundaries.
A comparative study has been started in the Wabigoon Subprovince in the western part of the Superior Craton of Canada. This project aims to: 1) apply multi-isotopic (U-Pb, Lu-Hf, O) analyses of zircon to map the lithospheric architecture in time and space; 2) determine if the distribution of mineral systems is controlled by this lithosphere architecture; 3) generate mappable exploration criteria for targeting various mineral systems at craton- to terrane scales.
The Wabigoon Subprovince can be subdivided into four terranes based on the whole-rock Sm-Nd isotopic data published by Tomlinson et al. (2004). Gold and copper mineralisation in the region appears to be controlled by the Winnipeg River and Western Wabigoon terrane boundaries (Fig. 1). However, the mineralisation within the Marmion and Eastern Wabigoon terranes does not follow the previously defined terrane boundaries (Fig. 1). In the Eastern Wabigoon Terrane, the assumed boundaries trend nearly E-W, whereas the gold and copper mineralisation forms a zone that trends northward. This discrepancy suggests that the terrane boundary may in fact strike northward (Fig. 2). Preliminary zircon Hf isotopic analysis shows that samples collected west of this hypothetcal boundary have older Hf model ages (3.5 Ga) than those from east of the boundary (3.1 Ga), suggesting that the western area has an older basement than the eastern area (Fig. 2). This difference in zircon Hf isotopes also suggests that the boundary of the East Wabigoon Terrane trends northward, which is consistent with the spatial arrangement of gold and copper mineralisation in the region. However, more data from both sides of this possible boundary are necessary to prove the case In the Marmion Terrane, the NE-trending mineralisation coincides with a NE-striking structure. There is contrasting in magnetic anomalies across this structure within the Marmion Terrane, which suggests that it is also a possible terrane boundary. The ongoing zircon mapping will test this hypothesis.
In summary, it appears that the spatial distribution of mineral systems (Au and Cu) in the Wabigoon Subprovince is controlled by the terrane boundaries (similar to the scenario in the Yilgarn Craton). The enhanced understanding of the interplay between lithospheric architecture potentially can help to bring about a paradigm shift in exploration strategy within the mineral industry, encouraging companies to use higher-precision multi-isotopic datasets to guide their area selection on the large scale.
This project is part of CCFS Themes 2 and 3, Earth Evolution and Earth Today, and contributes to understanding Earth’s Architecture.
Contacts: Campbell McCuaig, Yongjun Lu
Funded by: CCFS
Figure 2. Geology of Eastern Wabigoon area. The preliminarily analysed zircon samples are shown by stars. The blue stars represent samples with older Hf model ages of 3.5 Ga, whereas the green stars having younger Hf model ages of 3.1 Ga. The hypothesised terrane boundary is highlighted by a red thick dashed line.
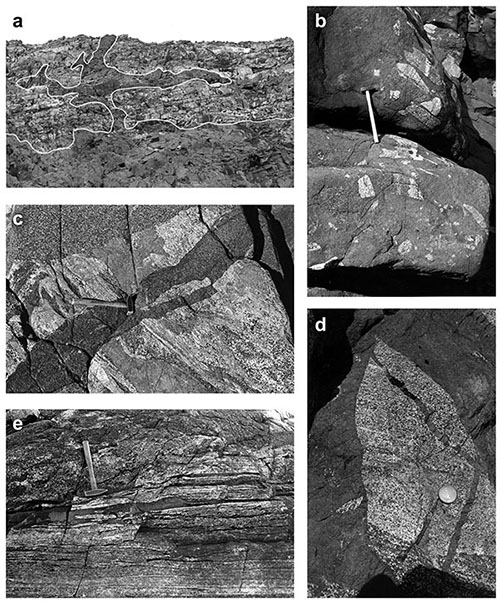
Cooking the lower crust: high-calorie ultramafic magmas in Arctic Norway
Most geologists are familiar with a wide range of mafic to felsic magmatic rocks, but the idea of ultramafic (high-Mg, low-Si) magmas is hard to assimilate, simply because laboratory experiments show that such magmas could only exist at temperatures that are not seen in the crust. Ultramafic lavas (komatiites) occurred widely in Archean time, but very rarely afterward; this has been used to argue for much higher mantle temperatures in the Archean. If ultramafic magmas do exist in the modern Earth, it would raise serious questions: how would they be generated, and how could they rise into the crust without crystallising?
Figure 1. Intrusive relationships. (a) Overview of the roof zone of the Nordre Bumandsfjord pluton, showing the contact between the dunite (massive grey) and the layered gabbros, which are intruded by the dunite along the contact, and by dunite sills and dikes. Length of photo ca 200 meters. (b) Intrusive breccia, with angular blocks of variably remelted layered gabbros in matrix of contaminated peridotite, which is cut in turn by a more massive dunite dike on the right side of the view; hammer shaft = 60 cm. (c) Xenolith of partially remelted gabbro with dunite sill along the layering is cut by a dike of contaminated peridotite; the whole xenolith is enclosed in a strongly contaminated, plagioclase-rich peridotite; hammer shaft = 30 cm. (d) Thin dunite dikes cutting a gabbro xenolith, enclosed in dunite; coin = ca 2 cm. (e) Massive dunite penetrating as sills along the layering of a strongly foliated gabbro near the eastern contact; hammer shaft = 30 cm.
The serendipity of a major continental collision, and the expansive exposures uncovered by a retreating icecap, the Caledonian nappe complex of Arctic Norway provides rare insights into the interaction between mafic-ultramafic magmas and the deep continental crust. The Kalak Nappe Complex contains >25,000 km3 of mafic igneous rocks, mostly layered gabbros, making up the 570-560 Ma Seiland Igneous Complex. The Complex has been intruded by a series of ultramafic magmatic rocks, including the Nordre Bumandsfjord Pluton. Field relationships in this pluton (Fig. 1-3) show that extremely fluid, dry, relatively Fe-rich (Fo81) dunite magmas intruded a pile of cumulate gabbros, with extensive block stoping and intrusive brecciation. Diking on scales from mm to meters, and extensive melting and assimilation of the gabbros, attest to high temperatures, consistent with a 2 km-wide granulite-facies contact aureole.
Figure 2. Anatectic phenomena. (a) Anorthositic dike, carrying xenolith of layered gabbro, crosscutting melted and strongly deformed gabbro; hammer shaft = 60 cm. (b) Bleaching at the rim of a gabbro xenolith, illustrating the removal of cpx-rich melts; note backveining of dunite by anorthositic melt issuing from rim of xenolith; ruler = 20 cm. (c) Wholesale melting of gabbroic xenolith (in peridotite visible at upper left), producing pegmatitic textures; hammer shaft =60 cm. (d) gabbro xenolith enclosed in contaminated peridotite, showing pegmatitic melting of xenolith rim; both xenolith and host peridotite are cut by dikes of less contaminated peridotite; hammer shaft = 60 cm. (e) Melting within gabbro xenolith, mimicking the pre-existing layering; gabbro is cut by dike of contaminated peridotite; pen = 15 cm.
Major- and trace-element trends show that the dunites were progressively contaminated by a clinopyroxene-rich partial melt of the gabbros, producing a range of lithologies from dunite through lherzolites to wehrlite. Experimental studies of natural samples at 0.8-1 GPa define the dunite solidus at 1650-1700 °C. In the average peridotite, contamination has dramatically lowered the solidus of the magma, producing a crystallisation interval of ca 400 °C (1600-1200 °C). This would provide large amounts of heat for melting and metamorphism, while the magmas remained fluid to relatively low T, consistent with the field relationships. Thermochemical and dynamic modelling shows that the dunitic primary magmas may represent the last melting of a rapidly ascending diapir of previously depleted subducted oceanic lithosphere. The mafic rocks of the Seiland Complex may already have been extracted from this diapir, and the late dikes of the province may reflect melting of the asthenosphere as the diapir spread out beneath the lithosphere. Ultramafic magmas, abundant in the Archean, may still be more common than usually assumed. However, they would only penetrate to the shallow crust under unusually extensional conditions, where ascent could outpace assimilation. See CCFS publication #237.
This project is part of CCFS Theme 2, Earth Evolution, and contributes to understanding Earth’s Architecture and Fluid Fluxes.
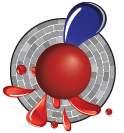
Contacts: Bill Griffin, Sue O’Reilly, Craig O’Neill
Funded by: TARDIS-E Foundation Project 2, CCFS
Figure 3. Contamination processes. (a) Dunite sills in heavily contaminated gabbro studded with fragments of residual anorthosite; scale ruler = 7 cm. (b) Residual clots of gabbroic plagioclase, scattered in heavily contaminated dunite; pen = 15 cm. (c) Heavily contaminated peridotite with folded schlieren of residual anorthosite, in sharp contact with relatively clean dunite, which appears to be intruded by the contaminated dunite; hammer shaft = 30 cm. (d) Ghost xenoliths, defined by residual plagioclase, enclosed in strongly foliated contaminated peridotite; pen = 15 cm. (e) Ghost xenolith of gabbro (10 cm diam.), defined by residual plagioclase, in moderately contaminated dunite. (f) Relatively homogeneous, strongly foliated and strongly contaminated peridotite, with relict knots of gabbroic plagioclase; hammer shaft = 30 cm.
Archean lower crustal rocks in southeastern Greenland: to hell and back 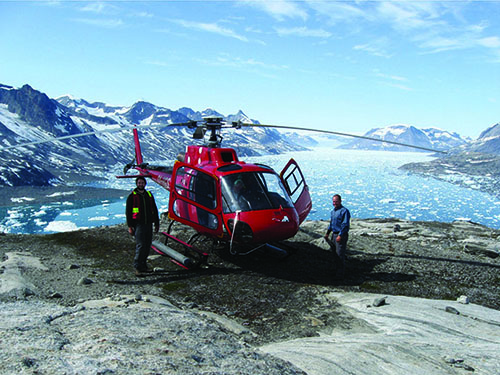
Ongoing work in CCFS suggests that many areas of relatively young crust are underlain by much older crust (CCFS publications #37, 38, 75, 95, 97, 163, 190), but the lower crust is rarely available for direct study. Much of the upper crust is commonly interpreted as originating from the partial melting of the lower crust, but there is considerable debate as to how these magmas are produced and move through the lower crust to their ultimate sites of emplacement, at shallower levels.
Figure 1. Field work in remote and rugged areas in southeastern Greenland relies on helicopter.
The Thrym Complex in southeastern Greenland appears to represent a very rare example of a section through the Archean crust, which includes ca 2750 Ma granulite-facies and ca 2700 Ma greenschist-facies rocks. It is made up of highly metamorphosed rocks representing major multiphase granitic bodies and a wide range of felsic, intermediate, mafic and ultramafic intrusions. These rocks are located in a high-alpine terrain at altitudes of around 3500 m above sea level (Fig. 1), and is one of the least studied regions of high-grade Archean gneisses and granulites in the North Atlantic Craton (if not in the world).
The CET is studying this remote region, in collaboration with the Geological Survey of Denmark and Greenland (GEUS), within the framework of the Foundation Project ‘Metal Sources and Transport Mechanisms in the Deep Lithosphere’ in the ARC Centre of Excellence for Core to Crust Fluid Systems (CCFS). This study took a regional approach in understanding the petrogenesis of the ca 2790-2700 Ma orthogneisses using new field data, petrology, whole rock geochemistry and U/Pb zircon age data on material collected in 2011. The principal aim was to understand the evolution of the early lower crust and to better decipher the regional geological history of the area.
Earlier studies have implied that the lower crust is predominantly mafic in nature. However, in the Archean lower-crustal section of the Thrym Complex, felsic gneisses predominate over mafic and ultramafic rocks.
The chemical and isotopic data suggest that the protoliths for the ca 2750 Ma orthogneiss in the Thrym Complex formed at the base of a tectonically thickened arc-like crust at temperatures between 800° and 1000 °C, and depths between 35 and 50 km. The mafic granulites and ultramafic rocks were metamorphosed at high grade, leading to partial melting with garnet and rutile present, consistent with the conditions expected in deep crustal levels or an arc root. With crustal thickening during continental collision, temperatures in this already hotter-than-normal crust can be amplified by increased radioactive heat production, leading to partial melting. This high-grade metamorphism was synchronous with similar metamorphism in western Greenland, suggesting that significant crustal thickening and possible relamination took place over much of the eastern part of the North Atlantic Craton in NeoArchean time (the Skjoldungen Orogeny; Fig. 2).
Our study of the Archean Thrym Complex in southeastern Greenland highlights the importance of integrating geochemical data with field observations in the development of geological models for high-grade gneiss terranes. For further information, see CCSF publication # 228.
This project is part of CCFS Theme 2, Earth Evolution, and contributes to understanding Earth’s Architecture and Fluid Fluxes.
Contacts: Leon Bagas, Marco Fiorentini
Funded by: CCFS, Greenland BMP, GEUS, CET, UWA
Figure 2. Model for the formation of the protoliths for gneisses in southeastern Greenland (from Bagas et al., Lithos 2013).
Subduction switches: geochemistry as a proxy for paleogeophysics in South China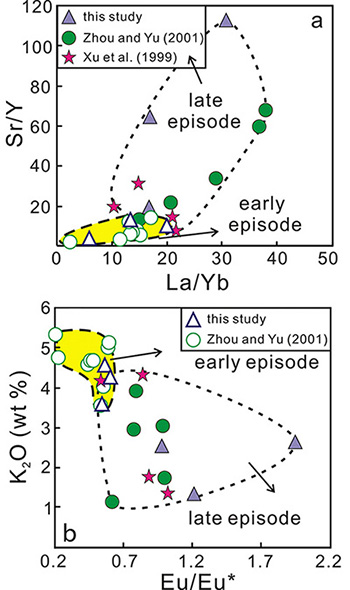
It is generally thought that late Mesozoic granite magmatism in the South China Block (SCB) shows an oceanward-younging migration. However, our new geochronological study of granites along the Pingtan-Dongshan Metamorphic Belt in the coastal part of the SCB, is not consistent with this trend. These late Mesozoic granites (and all those previously published) can be subdivided on their zircon U-Pb ages, into an early episode (194-140 Ma) and a later episode (140-66 Ma); the granites of the two episodes also have different geochemistry.
Figure 1. Geochemical differences between the early and late episode of late Mesozoic granites in the PDMB.
Both age groups of granites in this area are relatively depleted in Nb, Ta and Ti, but enriched in large-ion lithophile elements (e.g. Rb, Ba), with low Rb/Ba and Rb/Sr ratios and A/CNK values. These features are typical of I-type granitoids related to subduction. However, the early granites have higher Sr and K2O contents and higher La/Yb, Sr/Y and Eu/Eu* ratios than the later ones (Fig. 1), suggesting that the former originated from a shallower source with higher geothermal gradients, probably in a back-arc extensional setting, whereas the later ones derived from a deeper source with lower geothermal gradients; this probably was related to a compressional continental arc setting.
Integrating all recent precise zircon U-Pb ages, we find that the early episode of late Mesozoic igneous rocks (194-140 Ma) in the southeastern SCB formed in three pulses: 194-175 Ma, 174-151 Ma and 150-140 Ma. These pulses of magmatism mainly occur in the Nanling Range with EW-striking trends, probably extending eastward to the coast (Fig. 2). The granites of the later episode (140-66 Ma) mainly are found in the coastal SCB along a SW-NE trend. The early (140-100 Ma) magmatism is characterised by calc-alkaline I-type granites and probably formed in a continental-arc setting, whereas late (99-66 Ma) magmatism features alkaline A-type granites and occurred in an extensional setting.
The different distributions and tectonic settings of the late Mesozoic igneous rocks in the SCB contradict previous genetic models. Integrating our observations with previous studies on the subduction direction of Paleo-Pacific plate (Fig. 2), we propose that the early episode of late Mesozoic igneous rocks in the SCB were probably formed under extensional tectonics related to the northward subduction of the Paleo-Pacific plate in Jurassic time. In contrast, the granites of the later episode, running NE-SW along the coastal SCB, resulted from the NW-ward subduction of the Paleo-Pacific plate in Cretaceous time. This represents a major switch in the subduction direction. The oceanward-younging trend of the later episode probably was related to the rollback of the subducted plate.
This project is part of CCFS Themes 2 and 3, Earth Evolution and Earth Today, and contributes to understanding Earth’s Architecture.
Contacts: Qian Liu, Jinhai Yu, Sue O’Reilly
Funded by: NSF of China
Figure 2. Simplified new distribution and genetic model for late Mesozoic igneous rocks in the South China Block. The subduction of the Paleo-Pacific plate is inferred by Engebretson et al. (1985), Maruyama and Seno (1986) and Maruyama
et al. (1997).
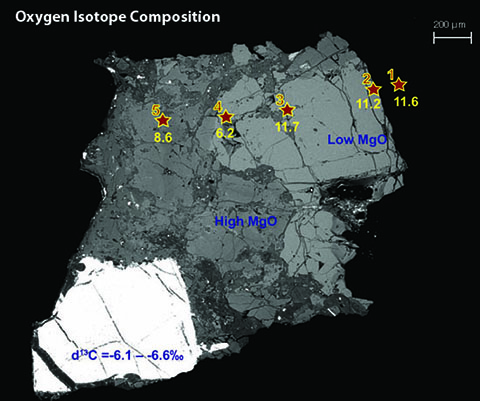
Unveiling mantle fluids using diamonds
Diamond-bearing eclogite xenoliths are common in the Udachnaya kimberlite, Siberia. Three types of garnet (Gnt) can be recognised in these eclogites, with different major-, trace-element and O-isotope compositions and related to different stages of mantle metasomatism.
Figure 1. Major- and TE- element composition of garnets from Udachnaya xenoliths.
The major- and trace-element compositions of garnets from 25 xenoliths show a well-defined change from positive to negative ratios of the heavy to light rare-earth elements (HREE/LREE) at a CaO content of 7.8-8.7%. This suggests either a change in fluid compositions or a change in garnet/fluid partitioning related to garnet composition. Both groups show similar trends of increasing MgO content in garnet due to mantle metasomatic processes. This is well evidenced by the difference in major-element, trace-element and oxygen isotope compositions between the original Garnet 1 and the metasomatic Garnet 2 in sample UE-12-2. Gnt1 and Gnt2 are usually found together with diamonds in metasomatic veins (Fig. 1). Garnet 2 forms Mg-rich rims on Garnet 1 (mg#=0.67-0.80 vs 0.57-0.58 for Garnet 1). The significant zoning in chemistry and its relationship to diamond with mantle-like carbon isotopes (δ13C =-5.6 - -6.6‰) suggest that Garnet 2 (and the diamond?) were produced by mantle fluids/melts shortly before eruption. Garnet 2 has a wide range in oxygen isotope composition (δ18O = 6.2-9.0‰), suggesting mixing of mantle fluids with the original isotopic system (Gnt1, δ18O =11.1-12.0‰). This pattern of decreasing δ18O with increasing mg# during metasomatism is similar to that identified in the Roberts Victor eclogite suite (see Research highligh p. 33-35).
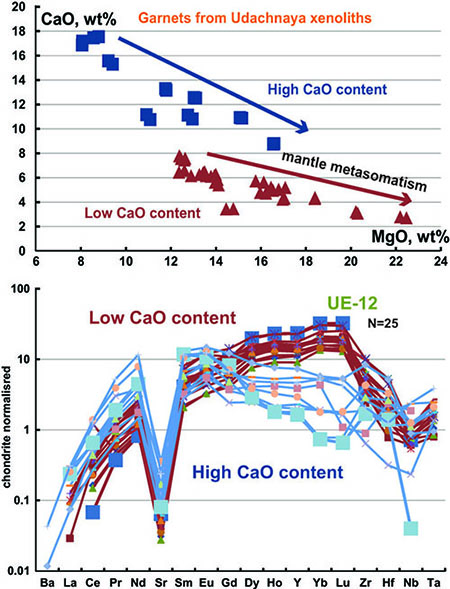
Figure 2. Oxygen isotope composition of Garnet 1 (original) and Garnet 2 (metasomatised); backscattered electron image of the sample (stars show traverse).
Garnet 3 is found in corundum-bearing eclogites and has much higher Ca (CaO=15.61-16.56 wt%) and different isotopic characteristics (δ13C =-3.6 - -5.3‰; δ18O =3.2-5.7‰). This suggests a different protolith, or a different metasomatic fluid from the one that produced Garnet 2. The Lu/Gd ratio increases significantly from Garnet (0.06-0.12) to Garnet 1 (4.0-4.2) to Garnet 2 (5.5-9.0). The demonstrated correlation between the oxygen composition of metasomatic garnet and high MgO contents, related to the formation of diamond with mantle-like carbon-isotope compositions, demonstrate the important role of mantle fluids. These fluids may also have been a trigger for the eruption.
This project is part of CCFS Themes 2 and 3, Earth Evolution and Earth Today, and contributes to understanding Earth’s Fluid Fluxes.
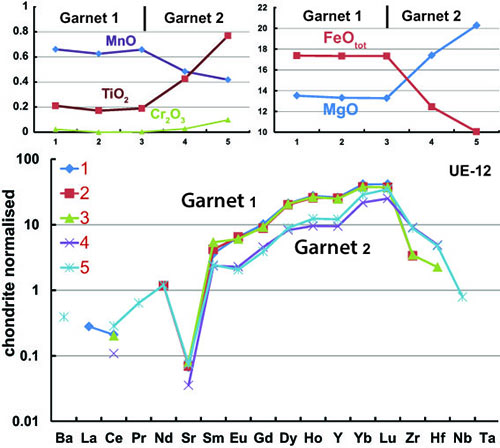
Contacts: Ekaterina Rubanova, Bill Griffin, Sue O’Reilly
Funded by: CoE CCFS Foundation Project “Diamond Genesis: Fluids in deep-earth processes, IPRS, MQ PGRF
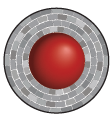
Figure 3. Changes in chemical composition of garnet across UE-12 sample.
Yilgarn dykes track details of supercontinent events: the transition from Nuna to Rodinia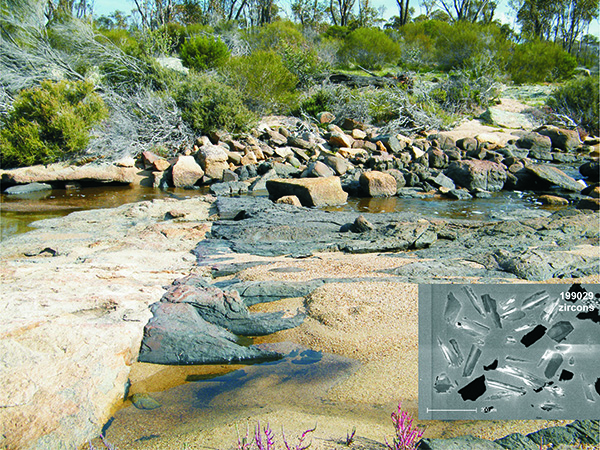
It has been widely accepted that the supercontinent Nuna (also known as Columbia) formed about 1800 million years ago (Ma) (see Research highlight p. 32-33 for more information about Nuna). However, precisely when it broke up, and how the resulting continental blocks reassembled to form the next supercontinent (Rodinia), is less certain. Australia held key positions in both supercontinents, and high-quality paleomagnetic data for Mesoproterozoic Australia are therefore crucial for understanding this process.
Figure 1. A northeast-trending, ca. 1.2 Ga mafic dyke (the dark outcrop) intruding granitic gneiss at the southeast margin of the Yilgarn craton. Inset shows zircon grains from the dyke. (Photographs by Michael Wingate)
The Gnowangerup-Fraser mafic dyke swarm is part of the Marnda Moorn large igneous province (LIP) and runs subparallel to the southern and southeastern margins of the Yilgarn Craton. Some dykes towards the craton margin are strongly recrystallised and others are deformed within the orogen, implying that at least some dykes were emplaced before the youngest deformation in the Albany-Fraser Orogen. Five dykes have previously yielded U-Pb ages between 1203 and 1218 Ma, and a positive baked-contact test suggests a that the magnetic remanence in the dykes is primary. The fossil record of past geomagnetic direction, which reflects the orientation and palaeolatitude of the continent at the time the rock formed, had been retrieved from a 1212 Ma Fraser dyke. To check this result and to get a true, time-averaged palaeomagnetic record, we collected samples from 19 dykes, along the Phillips and Fitzgerald Rivers, and north of Ravensthorpe. Stepwise demagnetisation provided a stable bipolar remanence from 14 dykes. A block sample from one of them, a coarse-grained dolerite was collected from the centre of a 20 m wide, vertical, northeast-trending (043°) dyke exposed in the Fitzgerald River (Fig. 1). The GSWA’s SHRIMP analysis of zircons from this sample indicated high and variable U and Th contents and Th/U ratios, typical of primary zircons in a mafic intrusion, and provided a preliminary crystallisation age of 1218 ± 6 Ma. A similar dyke, further east in the Phillips River, yielded a preliminary crystallisation age of 1211 ± 42 Ma, based on SHRIMP analyses of low-uranium baddeleyite.
The mean palaeomagnetic direction from the 14 dykes gives a palaeomagnetic pole at 55.8°N, 323.9°E, A95=6.5°, almost identical to the previously reported preliminary pole position from the 1212 Ma Fraser dyke. This robust paleopole, a rare key-pole for Mesoproterozoic Australia, places the West Australian Craton in a near-polar position at 1210 Ma (Fig. 2a). Comparison with coeval Laurentian paleopoles indicates that Laurentia and Australia would have been widely separated at that time (Fig. 2a). The two continents travelled very different paths between 1200 Ma and 1000 Ma (Fig. 2), and therefore could not have been parts of any supercontinent. This implies that the supercontinent Nuna must have broken apart before 1200 Ma, and Rodinia probably did not form until after 1070 Ma. Our concurrent geochemical analyses of this dyke swarm suggest a possible mantle plume connection for its formation. However, whether this swarm and coeval LIP events in other continents can be treated as parts of a single LIP to reconstruct palaeogeography, and how such plume event(s) are linked to supercontinent cycles, require further investigations.
This project is part of CCFS Theme 2, Earth Evolution, and contributes to understanding Earth’s Architecture.
Contacts: Zheng-Xiang Li, Sergei Pisarevsky, Xuan-Ce Wang, Michael Wingate
Funded by: CCFS foundation project 6, Detecting Earth’s rhythms: Australia’s Proterozoic record in a global context
Figure 2. Palaeomagnetically permissive palaeolatitudes of Australia and Laurentia at ca 1210 ma (a) and ca 1070 Ma (b), respectively, illustrating rapid changes in both palaeolatitudes and relative positions during the transition time between supercontinents Nuna (ca. 1800-1400 Ma) and Rodinia (ca. 900-700 Ma). The ca 1200 Ma key pole for Australia is from this study. The distinctively different apparent polar wander paths for the two continents (the thick bands with arrows, with colours corresponding their respective continents) suggest that the two continents travelled different paths during that time interval; in other words, they could not have been part of a supercontinent at that time.
Stealth attacks in Earth’s uppermost mantle: recognition of a new type of metasomatism and its consequences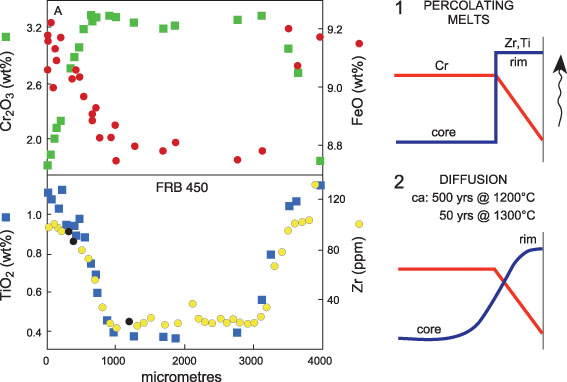
Mantle metasomatism is a relatively recent concept, introduced in the early 1970s when detailed studies of lithospheric mantle rock fragments (xenoliths), brought to the surface in basaltic to kimberlitic magmas, became widespread. Two main types of metasomatism were defined: modal (or patent) metasomatism describes the introduction of new minerals; cryptic metasomatism describes changes in composition of pre-existing minerals without formation of new phases. A new type of metasomatism has now been documented (CCFS publication #5), stealth metasomatism; this process involves the addition of new phases (e.g. garnet and/or clinopyroxene), but is a “deceptive” metasomatic process because it adds phases indistinguishable mineralogically from common mantle peridotite phases. The recognition of stealth metasomatism reflects the increasing awareness of the importance of refertilisation by metasomatic fluid fronts in determining the composition of mantle domains. Tectonically exposed peridotite massifs provide an opportunity to study the spatial relationships of metasomatic processes on scales of a metre to kilometers.
Figure 1. Zoning in a garnet from sheared lherzolite xenolith FRB450, and a model. (1) Instantaneous overgrowth of a garnet rim high in Zr and Ti, but low in Cr. (2) Diffusional modification of Zr and Ti profile. Chromium diffuses much more slowly, if at all (After Griffin et al. (1996)).
Mantle fluid types:
The nature of mantle fluids can be determined from the nature of fluid inclusions in mantle minerals and indirectly from changes in the chemical (especially trace-element) compositions of mantle minerals. Metasomatic fluids in off-craton regions cover a vast spectrum from silicate to carbonate magmas containing varying types and abundances of dissolved fluids and solutes. Fluid inclusions in diamond and deep xenoliths reveal the presence of high-density fluids with carbonatitic and hydro-silicic and/or saline-brine end-members. The data from deep cratonic xenoliths reinforce the importance of highly mobile melts spanning the kimberlite-carbonatite spectrum, which may become immiscible with changing conditions.
Figure 2. Inclusions of carbonatitic and silicic melts trapped in the Cr-diopside of megacrystalline lherzolite xenoliths from the A154 kimberlite, Slave Craton, Canada. Quench crystals of a Sr-rich carbonate and olivine show that these inclusions were molten at the time of kimberlite eruption, but that the melts had penetrated the pyroxene long enough before the eruption that the pyroxene was able to partially recrystallise and trap the melt inclusions. The lower two frames are mirror images at the same scale (After van Achterbergh et al. (2004)).
Effects of metasomatism on mantle geophysical properties:
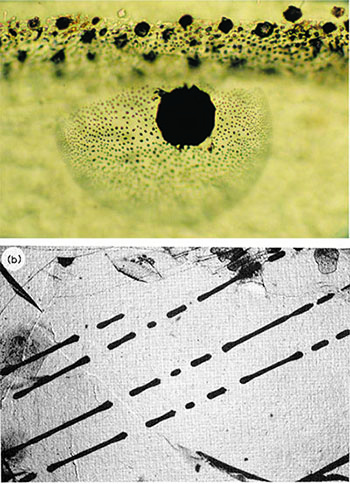
A critical conceptual advance in understanding Earth’s geodynamic behaviour is emerging from understanding the linkage between mantle metasomatism and the physical properties of mantle domains recorded by geophysical data. For example, metasomatic refertilisation of cratonic lithospheric mantle increases its density, lowers its seismic velocity and strongly affects its rheology. Introduction of radioactive elements (U, Th, K) increases heat production, and the key to understanding electromagnetic signals from mantle domains may be closely related to the distribution and type of fluids (e.g. carbonatitic, hydrous) and their residence in or between grains.
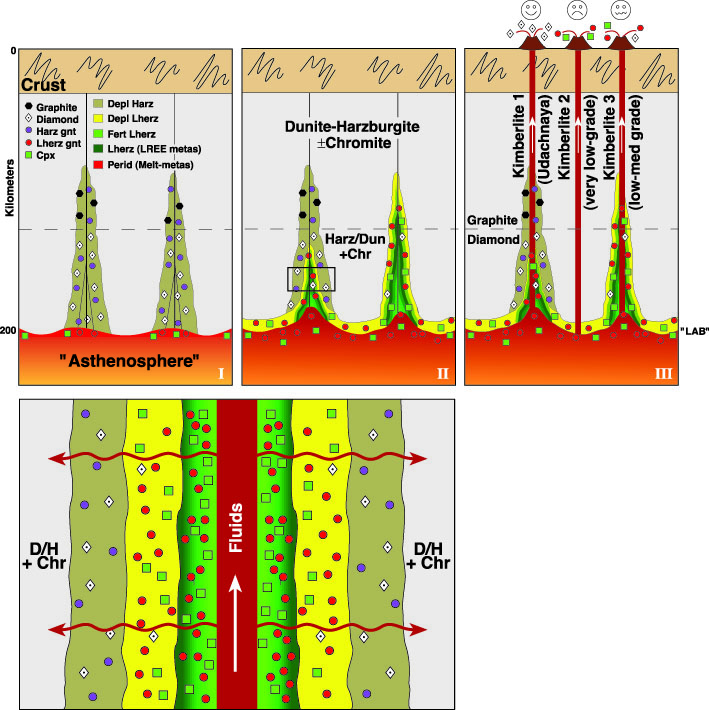
Figure 3. Photomicrographs of inclusions of sulfide melts, trapped in pyroxene from pyroxenite xenoliths from SE Australia. Left panel shows a single large inclusion that has expanded and fractured the pyroxene, sending molten sulfide and CO2 into the crack, which has recrystallised to trap a swarm of microinclusions. Right panel shows sulfide melts that originally were trapped as elongate hollow rods filled with liquid CO2, and then necked down to hollow spheres (After Andersen et al. (1987)).
Consequences of mantle metasomatism through time:
The lithospheric mantle is a palimpsest recording the multiple fluid events that have affected each domain since it formed. Metasomatism is the mechanism that primes mantle regions for metallogenic fertility (see Research highlight ) and recognition of metasomatic processes is providing a potentially global predictive framework for the location of some ore deposits (e.g. as Ni. Cr, Au, Cu, diamond) in the crust. These metasomatic events, involving different fluids and compositions, have repeatedly overprinted variably depleted original mantle wall-rocks. This produces a complex, essentially globally metasomatised lithospheric mantle, heterogeneous on scales of microns to terranes and perhaps leaving little or no “primary” mantle wall-rock. Decoding this complex record by identifying significant episodes and processes is a key to reconstructing lithosphere evolution and the nature and origin of the volatile flux from the deep Earth through time.
Figure 4. Cartoon illustrating a model for the early stages of cratonic metasomatism (After Malkovets et al. (2007)). (I) Primitive Archean SCLM, consisting of relatively oxidised harzburgite/dunite, is metasomatised by Si-bearing CH4-rich fluids brought in by low-degree melts from the underlying “asthenosphere”. Precipitation of diamond/graphite - harzburgitic garnet near fluid conduits. Melt-related metasomatism near the lithosphere-asthenosphere bound-ary (LAB) converts some harzburgites to lherzolite by addition of Ca, Fe, and Al. (II) Continued input of melts/fluids. Reduced harzburgite does not precipitate diamond/graphite. Melt-related metasomatism refertilises harzburgite to lherzolite at the base of the lithosphere and along conduits (weakly in left conduit, more extensively in right conduit). Relict harzburgitic diamonds in lherzolites. (III) Kimberlite eruption; high-grade pipes sample remnants of Stage-A modified mantle. Barren pipes sample least-metasomatised mantle and lack both harzburgitic garnets and diamonds. Some low-grade pipes sample highly metasomatised mantle with relict diamonds. The lower panel shows a detail of the melt conduit and the progressive metasomatism of the wall rocks, first by CH4-rich fluids expelled from the melts, and then by the melts themselves. Dun dunite, Harz harzburgite, Lherz lherzolite, Fert (re)-fertilised, Perid peridotite, Cpx clinopyroxene, Gnt garnet, Chr chromite, LREE light rare earth elements, metas metasomatised.
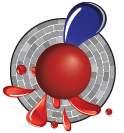
This project is part of CCFS Themes 2 and 3, Earth Evolution and Earth Today, and contributes to understanding Earth’s Architecture and Fluid Fluxes.
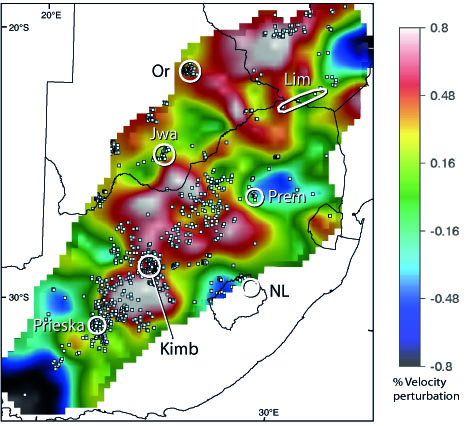
Contacts: Sue O’Reilly, Bill Griffin
Funded by: CCFS Foundation Projects 1 and 8, relinquished DP DP0984852
Figure 5. Detailed Vs tomography along a 1,000-km traverse at 200 km depth across the SW part of the Kalahari craton of southern Africa (Begg et al. 2009), with locations of kimberlites (Faure 2006). Circles and oval mark locations of well-studied xenolith and xenocryst suites. Lim Limpopo Belt, Prem Premier (Cullinan) Mine, NL northern Lesotho, Kimb Kimberley area, Or Orapa area, Jwa Jwaneng area, Prieska area lies across the craton margin. These suites clearly do not sample the highest-velocity (most depleted) parts of the
SCLM root.
Ancient reservoirs and recycled components: Linking plumes and plate tectonics
Whether mantle plumes and plate subduction are genetically linked is a fundamental question that impinges on our understanding of how Earth works. Earth’s materials are circulated between the surface and the bottom of the mantle through mantle-plume and plate-tectonic processes. The late Cenozoic basalt province in southeastern Asia is the first example that may demonstrate direct links between a young mantle plume and deep subduction. The presence of a young mantle plume rooted in the lower mantle is suggested by low-velocity seismic structures and a thinned mantle transition zone. Our pilot work, led by Dr Xuan-Ce Wang, has demonstrated that these young basalts were generated by partial melting of unusually hot mantle (CCFS publication #24).
Figure 1. Sr, Nd and Pb isotopes of late-Cenozoic least-contaminated LIS basalts from the Leiqiong area, the Indochina peninsula, and the South China Sea seamounts compared with Pacific MORB, south Indian Ocean MORB, and the Hawaiian OIBs. The fields of Pacific and south Indian Ocean MORB and the isotopic evolution of recycled components are also shown (for detail, please contact: Xuan-Ce Wang).
The synchronous less-contaminated basalts (εNd>+3) from the Leiqiong area, on the nearby Indochina peninsula, and the South China Sea seamounts (the LIS basalts) fall close to or within the range suggested for a FOZO (focal zone) mantle component, which is commonly proposed as a major component of the lower mantle (Fig. 1). The LIS basalts show a narrow range of 206Pb/204Pb, but large variations in 207Pb/204Pb, so they are bracketed by the 4.5- and 4.4 Gyr-old geochrons. This suggests the presence of a 4.5-4.4 Ga-old reservoir resulting from primordial differentiation of Earth mantle. This implies the presence of rocks that have been isolated from mantle convection currents since early in Earth’s history. These isotopic signatures are consistent with previous observations that argue for the existence of a plume, rising from the lower mantle in the Hainan region.
An important feature of the LIS basalts is the decoupling between isotopic and elemental signatures. The trace- and major-elements are highly correlated with trace-element ratios. With crustal contamination excluded, the correlations presented in Figure 2 suggest two end-member (high- and low-silica) melts. The high-silica end-member is similar to the bulk recycled oceanic crust and experimental melts of oceanic gabbro. The low-silica end-member melt compares well with the composition of incipient partial melts of garnet peridotite. However, there are no prominent correlations between elements and isotopes identified in this study. This suggests that the source of the late Cenozoic basalts may also contain young recycled components.
Figure 2. Covariation of selected trace element ratios and major elements with Sm/Nd ratios in the LIS basalts. The chondritic bulk silicate Earth (BSE), nonmodal batch partial melting (solid lines with square or circle) and binary mixing (dark blue dashed lines) between recycled oceanic crust (ROC) and peridotitic source derived melts are shown.
These conclusions have been confirmed by modelling of the isotopic evolution of recycled components (Figs. 1 and 3). First, the recycled oceanic crust may be the dominant factor controlling Pb isotopic heterogeneities in OIBs sources. Second, extremely high 187Re/188Os ratios recently reported in oceanic crust (80-675) would lead to very radiogenic 187Os/188Os ratios (187Os/188Os = 2-12) over 1 Ga (Fig. 3a), suggesting that such a component probably is absent in the LIS basalt source. Third, both ancient (>0.6 Ga) gabbro- and bulk oceanic crust-derived melts have distinctive Pb-Sr-Nd (Fig. 1) and Os (Fig. 3) isotopes that are significantly different from what we observed in the natural LIS basalts. Overall, our modelling results show that the maximum age for the recycled components in the source of the LIS basalts is <0.6 Ga.
Figure 3. Comparison of the Os-Pb isotopes of the Hainan basalts with isotopic evolution of different recycled lithologies.
These new findings, along with existing geophysical, petrological, geochemical evidence, confirm the coexistence of an ancient (4.5-4.4 Ga) mantle reservoir and young (0.2-0.5 Ga) recycled materials in the source region of the young Hainan plume. This study may provide the first observational support for dynamic linkages between deep subduction and the generation of mantle plumes (Fig. 4).
This project is part of CCFS Themes 2 and 3, Earth Evolution and Earth Today, and contributes to understanding Earth’s Architecture and Fluid Fluxes.
Contacts: Xuan-Ce Wang and Zheng-Xiang Li
Funded by: CCFS and NSFC
Figure 4. A cartoon illustrating the formation mechanism of the Hainan plume based on seismic imaging results and this study.
The end of the Archaean in the eastern Kaapvaal Craton: the 3.1 Ga Mpuluzi batholith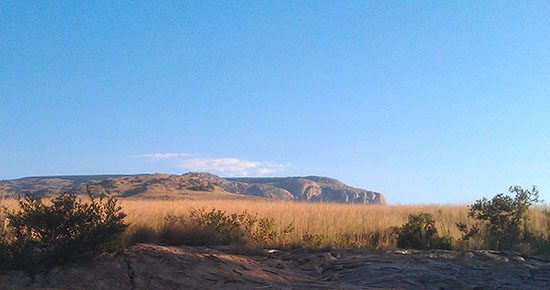
The Mpuluzi batholith forms part of the eastern Kaapvaal Craton in southeastern Africa - a piece of Archaean continental crust that has been stable for the past three billion years. The Mpuluzi outcrops over an area of approximately 2000 km2, and dominates the landscape, forming a high plateau that rises above the African grasslands (Fig. 1). The Mpuluzi is one of several large bodies of similar age in the region, along with the Nelspruit, Piggs Peak and Heerenveen batholiths, which total approximately 10,000 km2 (Fig. 2). These large granitoid intrusions were all emplaced at ~3.1 Ga, and are all unusual in form - they occur as 1-2 km thick sheets, rather than the deep-rooted batholiths common in Archean cratons. For half a billion years prior to their emplacement, the eastern Kaapvaal Craton was an active region, with large-scale melt emplacement events recorded at 3.51, 3.44 and 3.2 Ga. After the emplacement of the Mpuluzi and other 3.1 Ga granitoids, no major geological events were recorded in the region for another 600 Ma.
The emplacement of the Mpuluzi batholith, marking such a turning point in the history of the Kaapvaal Craton, is thus an event of significant interest in terms of crustal evolution processes. Although the older crustal remnants beneath the granite sheets - the Barberton Greenstone Belt to the northeast and the Ancient Gneiss Complex to the southeast - have been extensively studied, the Mpuluzi itself is still poorly understood, and there are few constraints on either the melt generation or emplacement chronology. This project has been designed to tackle some major questions: What was the heat source for the magmas, and what was the source material? How many pulses of magma were involved, and over what timescale? Why did emplacement occur in this unusual sheet-like form?
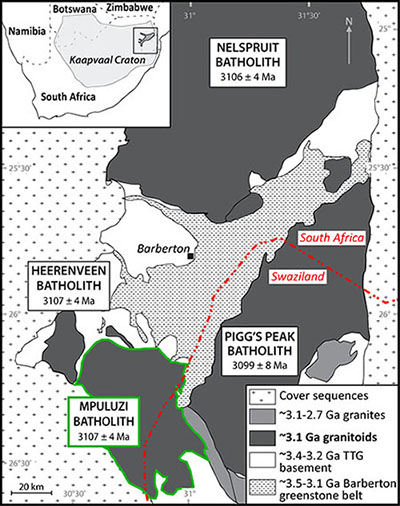
Dating the zircon from the Mpuluzi samples has proved to be a challenge, with many grains full of cracks and inclusions, leaving little ‘clean’ material to analyse. The high concentrations of the radioactive elements U and Th in the grains has meant that their 3 billion year life has been rather a hard one. Many have lost a lot of their radiogenic lead, as radiation damage progressively destroyed the crystal lattice.
The U-Pb ages obtained from Mpuluzi samples range from ~3.14 to ~3.09 Ga, with the main cluster of ages at 3.123 ± 7 Ga, emplacement may have occurred over a period as long as 50 million years. Some samples have a minor inherited population at ~3.5 Ga, which suggests that older crustal material was melted to produce the magmas. The Hf-isotope data provide further supporting evidence, with mean model ages of ~3.5 Ga, and in some samples extending back as far as ~4 Ga.
The Mpuluzi samples are currently being analysed for their Sr and Nd isotopic compositions, which will provide further constraints on the composition and age of the source material. The problems of the heat source and the unusual emplacement style will then be addressed through geodynamic modelling. Watch this space!
This project is part of CCFS Themes 2 and 3, Earth Evolution and Earth Today, and contributes to understanding Earth’s Architecture and Fluid Fluxes.
Contacts: Rosanna Murphy, Bill Griffin, Norman Pearson, Sue O’Reilly, Craig O’Neill
Funded by: CCFS Foundation Project, MQRES, EPS Postgraduate Fund
Metals in dirty water – the genesis of ore deposits 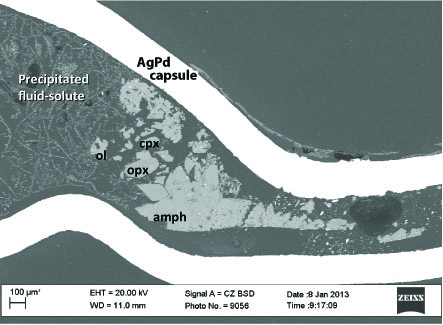
Field evidence shows that metal ore bodies are deposited by fluids derived from deeper in the Earth – but we do not yet understand in any detail how the metals are picked up from the deeper lithosphere and transported in the fluids. We have begun a multi-scale integrated study to address this void in our understanding. One part of the study involves a set of key experiments designed to evaluate the chemical behaviour of fluid systems at the conditions of the lithospheric mantle and the asthenosphere. The current focus is on the complex transport and concentration mechanisms of siderophile-chalcophile elements such as Ni, Cu and PGE in the deep lithosphere. Researchers from Macquarie and The University of Western Australia are collaborating on this Foundation Project, which addresses Theme 3 (Earth Today - and its Resources)
of the CCFS.
Figure 1. A longitudinal section of a capsule showing run products from a water-saturated experiment on a nepheline basanite at 950 °C and 2.0 GPa. The run products include solute precipitated from the quenched H2O-fluid (seen as filamentous strands of fine beads) and crystals of clinopyroxene, orthopyroxene, olivine, ilmenite, mica and amphibole.
We have conducted twelve experiments at 0.5-3.0 GPa and 950-1100 °C to determine the partitioning of minor and trace elements (including chalcophile metals) between hydrous fluids, peridotite minerals and typical intraplate basanitic melts. Five experiments were also conducted under H2O-undersaturated conditions on coexisting basanite and sulfide melts. The fluid/mineral/melt partitioning data, combined with previously obtained mineral/melt partition coefficients for the same basanite, provide information on the contrasting abilities of H2O-fluids and silicate melts to transport metals and incompatible elements within the mantle lithosphere. A particular feature of the experimental approach is that it avoids the use of fluid traps (including carbon spheres and fluid inclusions in solid minerals) commonly employed in similar experiments. This allows the experimental run products produced to be unambiguously identified (see Fig. 1) and analysed by electron microprobe and LA-ICPMS.
The successful experiments have produced a small but unique data set for H2O-fluids in equilibrium with mantle phases. There are very few equilibrium data on such very fluid-rich systems under these deep mantle conditions, so the data set is being carefully analysed. At 950-1100 °C and 2.0 GPa the fluids contain 15-25 wt % of dissolved solute. The solutes are enriched in SiO2 (56-66 wt %), Al2O3, and alkalis (10.9-12.6 wt % Na2O + K2O) but depleted in TiO2, FeO, MgO and CaO relative to the basanite. Overall the transport capacities of H2O-fluids within the upper mantle are distinctly different from those of silicate melts (Fig. 2). Alkalis, Pb and Ag are relatively enriched in the aqueous fluids, whereas most chalcophile and incompatible elements are not. As we continue to analyze these unique experiments, more partitioning data will be available on the economically important elements. Currently the results suggest the silicate melts can transport sulfides much more efficiently than aqueous fluids, but fluids may be critical in transporting metals such as Ag.
This project is part of CCFS Theme 3, Earth Today, and contributes to understanding Earth’s Fluid Fluxes.
Contacts: Marco Fiorentini, John Adam, Tracy Rushmer, Marek Locmelis
Funded by: CCFS Project 2a: Experimental determination of metal sources and transport mechanisms in the deep lithosphere
Figure 2. Mantle-normalised element concentrations in aqueous fluids and silicate melts equilibrated with a garnet lherzolite of Primitive Mantle composition. The fluid composition was calculated using partition coefficients from this study.
Decoding sulfur DNA solves how ancient ore deposits formed in Western Australia 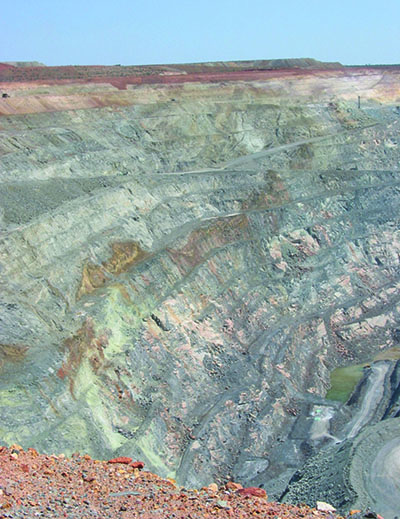
Magmatic hydrothermal oceanic vents represent places where metals accumulate in the form of exhalative and sedimentary sulfides associated with submarine felsic volcanoes. These are also loci where life can flourish in the form of a wide range of complex and diversified bacteria colonies. In the Archean Earth (more than 2.5 billion years ago), coeval to the emplacement of sulfide-bearing felsic magmas, komatiites locally erupted on the floor of the ocean. These hot and highly turbulent magmas assimilated previously formed volcanogenic exhalative and sedimentary sulfides, leading to the formation of discrete sulfide melts, which concentrated chalcophile and siderophile metals such as nickel, copper and the platinum group elements from the komatiite magma.
Figure 1. Exhalative sulfides in close spatial association with the largest komatiite-hosted nickel-sulfide deposit in the world, Mount Keith, Western Australia.
Multiple sulfur isotope data on sulfides from variably mineralised komatiite units in the Archean north Eastern Goldfields, Western Australia, provide new constraints on these assimilation and ore-forming processes. Although magmatic sulfides from komatiites display very similar Δ33S signatures to volcanogenic exhalative and sedimentary sulfides, they have consistently lower δ34S values relative to these sources. In other words, the sulfur-bearing compounds from the magmatic sulfides in the komatiites are on average isotopically lighter than the sulfur compounds contained in the volcanogenic exhalative and sedimentary sulfides. This lowering of the δ34S signature is consistent with degassing of the komatiite-sulfide melt system. At the temperatures and oxygen fugacities relevant to komatiite magmatism, sulfur in the melt exists primarily as 34S-poor sulfide species, while sulfur in the co-existing gas would be dominated by 34S-rich SO2. Continuous loss of this 34S-enriched gas would lower the δ34S values of coexisting sulfide melt, leading to magmatic sulfides with the isotopic compositions measured here.
Our results indicate that komatiites from the north Eastern Goldfields of Western Australia, irrespective of their initial sulfur content, degassed upon emplacement at Earth’s surface. Komatiite degassing likely influenced physical and chemical parameters of the primordial oceans by the addition of heavy sulfur in the form of SO2, thus contributing to the positive heavy S ocean signature. This then indirectly contributed to the creation of a complex chemical gradient at the interface between seawater and seafloor in the primordial Earth.
This project is part of CCFS Themes 1 and 2, Early Earth and Earth Evolution, and contributes to understanding Earth’s Fluid Fluxes.
Contacts: Carissa Isaac, Marco Fiorentini
Funded by: CoE CCFS Foundation Project “Early evolution of the Earth system and the first life, from multiple sulfur isotopes”; ARC Linkage LP0776780; SIEF postgraduate scholarship
Figure 2. Typical “komatiite country“ in the Eastern Goldfields of Western Australia.
Slaking the Earth’s thirst at mid-crust levels
Numerous studies have identified low-δ18O fluids in ductile shear zones that dissect volumes of otherwise anhydrous crustal rocks. A prime example is the shear-zone network that dissects the Proterozoic granulite terranes in central Australia. The pronounced lowering of δ18O values by up to 10‰ between rehydrated fault-zone rocks and their adjacent largely anhydrous equivalents precludes the involvement of internally-derived fluid sources. This is problematic because these shear zones typically post-date the high-grade regional metamorphism of their wall rocks by tens or hundreds of millions of years. As a consequence, in cases where calculated fluid compositions are below the mantle signature (δ18O = 5.7 ± 0.3‰), such alteration patterns are typically interpreted as the product of deep crustal metasomatism driven by the influx of surface-derived fluids. However, models that propose the migration of a mobile fluid phase from the surface to the mid-crust are both mechanically and geochemically challenging.
We have used the Cameca 1280 ion microprobe at the University of Western Australia to analyse oxygen isotopes in garnet porphyroblasts from the mid-crustal Walter-Outalpa shear zone, southern Curnamona Province, South Australia. All the garnets have homogeneous δ18O values of < 3‰. Integrated Lu-Hf geochronology and compositional mapping by electron microprobe demonstrate that the closed-system growth of these isotopically light garnets (Fig. 1) started as early as 531 Ma, prior to the peak of metamorphism and deformation during the Delamerian Orogeny (514–490 Ma). These new data have lead to the proposition that the prograde burial and dehydration of altered fault panels under thick sedimentary sequences during pre-orogenic basin formation has produced the observed lowering of the δ18O values. This contrasts with established
fluid transport models, where surficial fluid signatures are imposed at depth by large fluxes of downward-penetrating fluids. The existence of low δ18O values in deeply-exhumed shear zones may therefore indicate that the fault structures had a pre-metamorphic history of near-surface exposure, weathering, burial/metamorphism and re-exposure.
This project is part of CCFS Theme 2, Earth Evolution, and contributes to understanding Earth’s Fluid Fluxes.
Contacts: Chris Clark, John Cliff
Funded by: ARC Discovery
Figure 1. Major element chemical, O-isotopic and Lu-Hf geochronological dataset from a single garnet from within the Walter-Outalpa shear zone in central Australia (Raimondo et al., 2013, Geology, in press).
Unravelling the early Earth’s record of biological fingerprints using sulfur isotopes 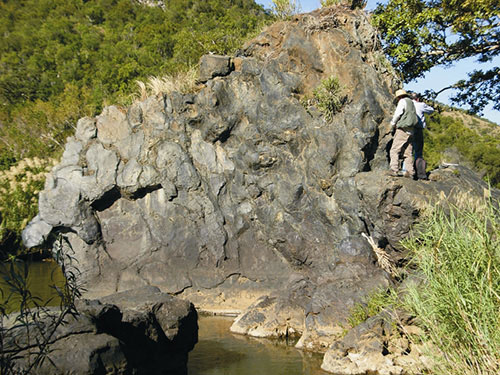
Multiple sulfur isotopes (32S, 33S, 34S, 36S) are becoming an increasingly important tool to investigate biological processes on the early Earth. They can tell us about the types of life present in Earth’s earliest sedimentary environments and trace the transfer of sulfur species in fluids and gases from the interior of the Earth via the atmosphere, hydrosphere, and finally into the biosphere.
One problem in this type of study is the spatial resolution at which multiple sulfur isotopes are currently analysed. Traditional bulk analyses can lead to artificial homogenisation of sulfur-isotope signals in a sample and conceal evidence of processes on the micron scale (the scale where microbial mediation might be observed). Our work under the CCFS Theme: The Early Earth is using in-situ techniques (SIMS and NanoSIMS), where the spatial context of the analyses is retained, to gain new insights into this problem.
Using the CAMECA NanoSIMS at UWA in the past year, we have been able to extract sulfur-isotope data (δ34S) from tiny (<15 μm) pyrite grains within microtubes in ca 3.5 billion-year-old basalts. These record δ34S values as low as ca -40 ‰, indicating that sulfur processing microbes were a likely component of the early Archean sub-seafloor biosphere (CCFS publication #230).
3.5 billion-year-old pillow lavas from the Barberton greenstone belt, South Africa, containing microtubes with microbially-mediated sulfide inclusions.
We have also used the CAMECA IMS1280 (see Technology development section of this Report) to analyse some of Earth’s oldest sedimentary sulfides from the 3.5 billion-year-old Dresser Formation of Western Australia. Here we have measured all 4 stable sulfur isotopes, and while the δ34S and Δ33S values appear to be similar to previous bulk analyses, the Δ36S values have a much greater spread than previously reported. This could be significant for understanding the sources of sulfur on the early Earth, but more work is needed to test the robustness of SIMS Δ36S data. As a result we are now collaborating with international experts such as James Farquhar (University of Maryland) to conduct further tests on these samples using both SIMS and more traditional techniques.
We are also implementing modern analogue experiments to discover the micron-scale distribution of multiple sulfur isotopes in microbially-precipitated sulfides. Here we are working in collaboration with microbiologists and isotope geochemists in Norway and USA, using cultures of different sulfur-processing microbes, in particular sulfate-reducers and sulfur-disproportionators. The isotopic compositions of the end products of these experiments will soon be analysed using SIMS and NanoSIMS. It is hoped that this will give us more accurate interpretations of Archean sulfur isotope data and help us understand the anomalous Δ36S signatures seen in our data from the Dresser Formation.
This project is part of CCFS Theme1, Early Earth, and contributes to understanding Earth’s Fluid Fluxes.
Contacts: David Wacey, Mark Barley
Funded by: ARC CCFS CoE
Nanoscale secondary ion mass spectrometry δ34S sulfide data from ~3.5 Ga Hooggenoeg Formation South Africa shows vein sample (red), two candidate biotexture-bearing samples (green), and standard (blue). (a): Analyses in order performed: B137 ranges from +6.7‰ to +18‰ and is positively shifted from CMCA-S1 pyrite standard; B175 and B177 are negatively shifted from standard, with B175 ranging from −27.3‰ to −3.2‰ and B177 from −39.8‰ to −8.3‰ (error bars are 2σ). (b): Range in measured δ34S values compared to other Archean sulfides. Candidate biotexture-bearing samples show largest range and most negatively shifted values yet measured, whereas vein sample is positively shifted and shows smaller range. For reference, yellow band indicates mantle sulfur, gray line indicates Archean seawater, and purple line (HT) indicates hydrothermal sulfides (from Mojzsis, 2007). For comparison, δ34S range of sulfides from cherts and sediments of similar age are shown: 3.49.
The internal heat of the Earth drives plate tectonics, so the surface heat flux can give us an important constraint on the properties and behavior of the Earth through geologic history. The surface heat flow can be reliably measured over continents, but due to hydrothermal circulation in oceanic crust, measurements in ocean basins often do not reflect deep lithospheric heat flow. As such, estimates of lithospheric heat flow over ocean basins usually are based on geodynamic models. These models usually are simple, using constant thermal properties and boundary conditions – but are they useful?
Figure 1. Predicted and measured seafloor heat flow. The thick blue line is our preferred model. The dashed red line is a conventional plate model, and the data points and dashed violet lines describe global heat flow data.
We have developed new thermal models that incorporate a petrologically and thermodynamically more complete view of lithospheric cooling. These models show that several thermal complexities are important as they change the predicted surface heat flow over ocean basins significantly while remaining compatible with reliable measurements. Specifically, we have shown that the effective thermal properties of the oceanic lithosphere depend strongly on age, and that this age-dependence is primarily due to the thermal insulation effect of oceanic crust. Therefore, heat flow over young seafloor is significantly lower than conventional models predict while predictions at older ages are similar.
This revision of conventional thermotectonic theory has several major implications. For example, lower heat flux over young seafloor indicates that the advective or hydrothermal component of heat transport has been significantly overestimated. This changes the hydrogeological regime of oceanic lithosphere and impacts on geochemical mass-balance problems related to the fluid interaction of lithosphere and oceans. Also, strongly age-dependent cooling affects the seafloor subsidence rate, and in this case our models are consistent with global topographic data. This shows that persistent features of lower magnitude than roughness in the data can be identified. In addition, the modeled net seafloor heat flow, and thus global heat flow, is lower than conventional models predict. Since the low conductivity of oceanic crust is the principal reason for the low heat flow over young seafloor, this effect is expected to be more important early in Earth history when the oceanic crust was thicker than it is now. This means that seafloor heat flow in the Archean may have been significantly lower than conventional models predict, or that plate velocity would have to be much higher to compensate for low-conductivity lithosphere.
We also have coupled our revised thermotectonic model of oceanic lithosphere to an isostatic model of the Earth to predict eustatic sea level changes over Earth history. Remarkably, our model predicts systematic sea-level change in good agreement with the Phanerozoic record, and we calculate that sea level at the end of the Archean (2.5 Ga) was at least 2 km higher than it is now, although our estimates are highly dependent on the choice of melting model. Ongoing research in this project will refine these predictions.
This project is part of CCFS Theme 3, Earth Today and contributes to understanding Earth’s Architecture.
Contacts: Chris Grose, Juan Carlos Afonso
Funded by: ARC Discovery (JCA); ARC IPRS
Figure 2. Predicted sea level and thickness of oceanic crust since the start of the Archean. The red line shows data for Phanerozoic sea level (Hallam, Phanerozoic sea-level changes, Columbia Univ. Press, 224p, 1992).
Squeezing the mantle: between an ocean and a continent
Tectonically-driven deformation of the lithosphere leads to strain localisation through the formation of ductile shear zones, and their development is responsible for lithosphere-scale deformation that controls the nature and distribution of Earth’s tectonic plates. Divergent plate boundaries, such as ocean-continent transitions, are the perfect place to study mechanisms activated during extension leading to localisation of deformation. The Platta-Totalp massifs in the Eastern Central Alps (Grison, Switzerland) are a type example of a zone of exhumed continental mantle. Mapping and structural analysis of the nappes has produced a palinspastic reconstruction of a complete stratigraphic sequence of an ocean-continent transition (Fig. 1) that can guide sampling for a study of deformation processes.
Figure 1. Palinspastic reconstruction of the Platta nappe (Desmurs et al. 2001, Geol Soc London Spec Publ). (a) Pictures showing the present observed Alpine structure. (b) Palinspastic section across the Platta-Err ocean -continent transition. It shows the position of the upper and lower serpentinite units within the former ocean-continent transition and summarises the field relationships between fault structures, continental rocks, exhumed mantle rocks and intrusive and extrusive mafic rocks (Schaltegger et al. 2002, Terra Nova).
The mantle rocks in these units are spinel lherzolites and harzburgites, into which gabbros and basaltic dykes were intruded (Fig. 2a). Mantle rocks close to the continent are spinel peridotite mixed with (garnet)-pyroxenite layers while the ultramafic rocks at some distance from the continent are pyroxenite-poor peridotite that equilibrated in the plagioclase stability field (Fig. 1). Two-pyroxene and single-orthopyroxene thermobarometric data from the Platta nappe show that the equilibration temperature (at 25-45 km depth) increases from 850±50 °C near to the continent to >1000 °C further oceanwards (Desmurs, 2001, PhD ). The change in temperature is related to the exhumation of the massif during crustal thinning. Some peridotites contains garnet pyroxenite, indicating high-pressure formation within the spinel peridotite field (>45 km depth) in the mantle sequence.
Figure 2. (a) Ophicarbonate at the top of the mantle sequence; (b) Peridotite with foliation in the upper serpentinite unit; (c) Variation of the peridotite composition in the upper serpentinite unit.
Fieldwork in the upper mantle part (Fig. 1) reveals heterogeneous distribution of deformation from lower to upper serpentinite units. The peridotite is deformed in both units, but is more deformed in the upper serpentinite unit (Fig. 2b). At a smaller scale, the deformation and the composition of the rock are heterogeneous. The peridotite shows dunitic and harzburgitic layers, and high-temperature shear zones at metre or centimetre scale (Fig. 2c and 3). Sampling across this sequence gives a spatial resolution of the deformation within an ocean-continent transition. A detailed study of the peridotite texture is being combined with the analysis of the crystallographic preferred orientation of minerals, using electron backscatter diffraction method (EBSD) to determine the deformation mechanisms activated during extension. This study is coupled with thermometric calculations to constrain the temperature of the deformation. The study will constrain the localisation of the deformation related to decreasing temperatures and possible percolation within extensional settings such as ocean-continent boundaries.
This project is part of CCFS Themes 2 and 3, Earth Evolution and Earth Today, and contributes to understanding Earth’s Architecture and Fluid Fluxes.
Contacts: Mary-Alix Kaczmarek, Steve Reddy
Funded by: CCFS and Curtin University
Figure 3. Crossed-nicol photomicrograph of an ultra-myloniticperidotite.
Multistage refertilisation of an Archean peridotite massif (NE Tibet, China)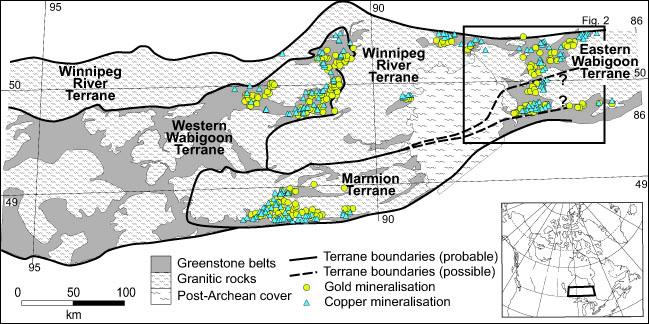
Peridotite massifs in orogenic belts and mantle xenoliths brought up by volcanic rocks both reveal the lithological and geochemical heterogeneity of the subcontinental lithospheric mantle (SCLM). Pyroxene-rich veins, secondary metasomatic phases and elemental and isotopic enrichments of Ca-, Al-rich minerals are common in orogenic peridotites. These fertile markers within the depleted host rocks suggest a complicated history of refertilisation. To sort out the sequence of multistage refertilisation in the SCLM is tricky, because of the overlapping of enrichment events and the rarity of useful dating targets. However, a study of elemental and isotopic (Sr-Nd-Hf-Os) compositions of minerals of the Shenglikou peridotite massif from the North Qaidam orogen (NE Tibet, China; Fig. 1) has revealed unusually clear details of the refertilisation history.
Figure 1. Tectonic units of the Northeastern Tibet area, China. The garnet peridotite massif is enclosed by ultrahigh-pressure (UHP) metamorphosed gneiss in the Shenglikou area, located at the western segment of the North Qaidam orogen. This orogen also exposes UHP rocks at the Yuka, Xitieshan and Dulan areas.
This ultramafic massif was scraped off from the Qilian continental-margin mantle wedge by the subducting Qaidam block during early Paleozoic assembly of the Tibetan plateau. Refractory dunite and harzburgite formed the original lithologies, which enclose fertile garnet lherzolite zones, secondary clinopyroxene-rich lherzolite/wehrlite layers (Fig. 2) and rare garnet pyroxenite dykes. Re-Os isotopic analyses of Fe-Ni-sulfides from the peridotite give some ancient Re-depleted model ages (oldest ≈ 3.0 Ga), suggesting an Archean origin. Whole-rock oxide compositions show a linear mixing trend between the dunite and pyroxenite. Trace-element patterns imply the dunite-harzburgite protoliths were re-enriched by slab-derived fluids, and the lherzolite and wehrlite look like the products of reaction between the refractory peridotite and a pyroxenite melt with an arc signature.
Figure 2. Clinopyroxene-rich garnet lherzolite/wehrlite (light green) inter-banded with garnet harzburgite host (dark green). The exposed pen is about 10 centimetres.
However, mineral elemental and isotopic data argue against a single refertilisation by binary mixing. The initial Nd-Hf isotopic patterns (Fig. 3) indicate that the dunite-harzburgite protoliths, clinopyroxene-rich peridotites and pyroxenites record different isotopic evolution paths. Their Hf and Nd isotopes, compared with chondrite and depleted-mantle values, suggest that the Hf isotopes can record ancient events and are more immune to later metasomatism, but the Nd isotopes were largely shifted to less radiogenic values (Fig. 3) by subsequent enrichment events. Lu-Hf analysis of garnet and clinopyroxene from refractory garnet harzburgites away from the refertilised lithologies give an isochron age around 1.5 Ga (Fig. 4), consistent with their Hf depleted-mantle model ages; this suggests an early Mesoproterozoic refertilisation of this piece of Archean mantle. Garnet- and clinopyroxene-rich rocks (secondary layers and pyroxenite dykes) show Sm-Nd and Lu-Hf isochron ages of ~1.1-0.6 Ga. This time span may imply a refertilisation event at ~1.1-0.9 Ga (Xiong et al., 2010) that formed the secondary peridotite layers; then pyroxenite melts intruded the refertilised mantle before the incorporation of this mantle fragment into the subducting slab at ~430 Ma. Radiogenic Sr (87Sr/86Sr initial=0.71266-0.71388), unradiogenic Nd isotopic data but near-DM Hf isotopes (Fig. 3) suggest the melt came from the asthenosphere, which nad been metasomatised by slab-derived material
.
Figure 3. 143Nd/144Nd initial versus 176Hf/177Hf initial ratios of garnet, clinopyroxene and amphibole in different lithologies of the Shenglikou peridotite massif. Nd and Hf isotopic ratios are calculated back to 429 Ma, when the zircon formed in this massif by UHP metamorphism. Error bars are shown as ±1σ, and those of clinopyroxene and amphibole are smaller than the symbol size. “S L”, secondary lherzolite; “H”, harzburgite; “DM”, depleted mantle; “CHUR”, chondrite uniform reservoir; “Gt”, garnet; “Cpx”, clinopyroxene; “Amp”, amphibole.
This work has shown that different incompatibilities and activities of isotopic pairs in rock-melt or rock-fluid systems can reveal different mantle events, and help us to clarify the sequence of multistage refertilisation in a volume of ancient SCLM.
This project is part of CCFS Theme 2, Earth Evolution and contributes to understanding Earth’s Architecture and Fluid Fluxes.
Contacts: Qing Xiong, Sue O’Reilly, Bill Griffin, Norman Pearson, Jianping Zheng (China University of Geosciences, Wuhan)
Funded by: ARC Discovery and Centre of Excellence (S.Y.O’R and W.L.G.), NSFC (J.P.Z.)
Figure 4. 147Sm/144Nd present versus 143Nd/144Nd present (a) and 176Lu/177Hf present versus 176Hf/177Hf present (b) diagrams of garnet, clinopyroxene and amphibole in different lithologies of the Shenglikou peridotite massif. Error bars are shown as ±1σ, and those of clinopyroxene and amphibole are smaller than the symbol size. Isochron ages (±2σ) are shown; the large uncertainties reflect the measurement of Lu/Hf ratios by LA-ICPMS and will be improved by further work. Abbreviations and symbols are the same as those of Figure 3.
Spurious Hadean ages in East Antarctica: the tribulations of moving Pb
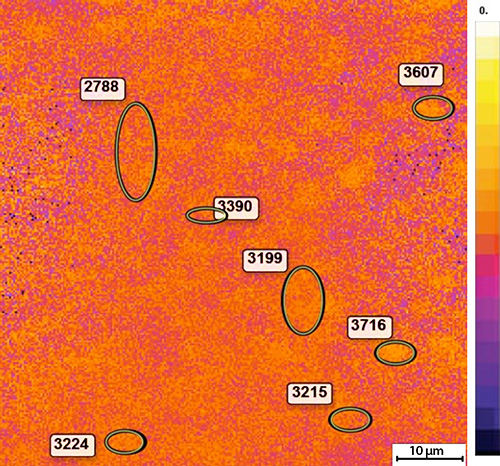
Zircons from early Archean ortho- and paragneisses in the ultrahigh-temperature (UHT) metamorphic rocks of the Napier Complex of East Antarctica show very complex U-Th-Pb systematics. Published ages from the Mount Sones, Dallwitz Nunatak and Gage Ridge localities are scattered, and the oldest ages are reversely discordant (U/Pb ages older than 207Pb/206Pb ages). This problem attracted scientific attention several years ago, because 207Pb/206Pb ages are considered to be more robust than U-Pb ages in ancient rocks. Several different mechanisms were proposed to explain this phenomenon, but no satisfactory answer was forthcoming. Since uranium is strongly lattice-bound in zircon, it is unlikely to be mobilised, so the favoured explanation was “Pb gain”, a mechanism opposite to Pb loss in normally-discordant data. However, the source of the “extra Pb” was not defined, and this problem has remained unresolved for more than 25 years.
Figure 1. Scanning ion images of grain n3852-08 from Mount Sones sample 14178-2 using a single-collector routine: (a) 48Ti, (b) 89Y, (c) 180Hf, (d) 206Pb.
We have used a novel high spatial resolution ion microprobe imaging technique to investigate the problem (CCFS publication #276). Selected areas of 70 μm x 70 μm of Antarctic zircons were imaged using a small (~2 μm) rastered primary beam on the Cameca IMS 1280 at the National History Museum in Stockholm. The distribution of 48Ti, 89Y, 180Hf, 206Pb, (Fig. 1), 232Th and 238U was imaged in the single-collector mode and 204Pb, 206Pb, 207Pb and 180Hf in the multi-collector mode. Hafnium is evenly distributed in these zircons and served as a proxy matrix peak in both routines.
A zircon grain from a paragneiss from Mount Sones with a spot age of 3008±21 Ma (207Pb/206Pb age) and U content of 2561 ppm was one of those selected for investigation. The ion maps (Fig. 1b) reveal the distribution of selected elements. Cathodoluminescence (CL) images show that Yttrium, Th and U define a zonation. The Pb distribution, in contrast, shows an unusual patchiness (Fig. 1d). Although it broadly follows the U and Y zonation, there are bright patches with enhanced signals which do not correspond to any zones or to crystal imperfections (e.g. cracks). Ti also shows patchy distribution, but there is no direct correlation between patches of Pb and Ti.
In the multicollector mode, 206Pb and 207Pb isotopes exhibit similar patchiness. The 204Pb image (not shown) is black, showing there is common Pb in the analysed area. Using the WinImage program, we produced maps of 207Pb/206Pb (Fig. 2), and these maps allow calculation of 207Pb/206Pb ages for spots of any size from ~2 μm2 upward, within the frame of the picture (70 μm x 70 μm) and at any time after data collection. This is a new and unique method for obtaining age information from zircon, and new applications await.
These maps show areas of enhanced brightness where the 207Pb/206Pb ratio is higher and demonstrate that within these small areas (μm scale) the apparent 207Pb/206Pb age is older than in the rest of the crystal. Using these images, we have calculated 207Pb/206Pb ages ranging from 2.8 Ga up to 3.7 Ga (Fig. 2), both older and younger than the apparent SIMS age. Spuriously old 207Pb/206Pb ages in areas enriched in radiogenic Pb reflect a combination of supported and unsupported radiogenic Pb; Pb has indeed moved, but only within the grain, so there is no reason to invoke “extra Pb”. In addition, the ‘patchy’ distribution of Ti has the potential to affect Ti-in-zircon thermometry, with implication for the accurate determination of zircon crystallisation temperatures.
Some of the ages measured in this zircon suite are Hadean (>4 Ga) and hence Pb redistribution of this type might have lead to the rocks being classified as samples of Earth’s oldest crust. In the present example, the mobilisation and redistribution of Pb in Antarctic zircons probably occurred at ~2.5 Ga, the time of UHT metamorphism in the Napier Complex. This well-documented event reached temperatures >1100 °C, the highest recorded in Earth’s continental crust.
Figure 2. Multi-collector map of 207Pb/206Pb. Ellipses show the areas used for 207Pb/206Pb age calculation. Field of view is 70 μm x 70 μm.
All images are normalised to the HfO image to minimise the effect of enhanced ion emission from the original spot analysis crater. The colour-scale bars are relative intensity (i.e. do not correspond to ppm).
This project is part of CCFS Theme 1, Early Earth and contributes to understanding Earth’s Architecture.
Contacts: Monika Kusiak, Simon Wilde
Funded by: EU-FP7, Marie Curie Grant: CCFS Foundation Project 2
Glittering gold nugget!
Gold is a highly siderophile element (HSE) along with the platinum group elements (PGEs: Pt, Pd, Rh, Ru, Os and Ir) and Re. HSEs are elements that strongly prefer the metallic phase in comparison to silicate phases, and therefore are important tracers for differentiation events in Earth’s history during the formation of the metallic core. However, our knowledge of gold abundance and its distribution in the mantle is limited. In this study, Au has been analysed along with major- and trace-elements in sulfides in fragments of the Earth’s mantle (xenoliths) brought to the Earth’s surface in basaltic magmas that formed Quaternary volcanoes in the Svalbard Archipelago (between north Norway and the North Pole). Combining these data with silicate data allows us to build a model of Au behaviour during mantle processes.
Analyses of the trace elements in clinopyroxene grains from the peridotite (olivine-rich) mantle xenoliths reveals there are three different types as seen by the contrasting patterns in Figure 1.
There is an increase in the light rare-earth (LREE) elements progressing from Group I to Group II to Group III. This type of change in pattern can be due to the changing composition of fluids infiltrating the mantle rocks. These fluids change the composition of minerals in these rocks (metasomatism), and also progressively change their own composition through two-way reaction, a process similar to that in a chromatographic column. The strong enrichment in LREEs and Sr coupled is accompanied by a decrease in Ti and suggests that the metasomatic agent was a carbonatitic melt or fluid.
Locality maps: left shows the sample localities, right shows the broader geological context of Svalbard.
Sulfide grains are the residence site for platinum group elements in mantle rocks. Three distinct patterns for PGEs have been observed in the Svalbard sulfides (Fig. 2). The PGEs can be divided into two groups: the PPGEs (Pt, Pd and Rh), which are incompatible and therefore removed during melting, and the IPGEs (Ir, Os and Ru), which are left behind with the residue during mantle melting. Type 1 sulfides are residual sulfides, with average (PPGE/IPGE)n = 0.53; Type 3 sulfides were introduced during metasomatism with average (PPGE/IPGE)n = 3.61; Type 2 sulfides fall in between with average (PPGE/IPGE)n = 0.80. The Type 2 sulfides represent a mixing between a residual sulfide and the metasomatic agent that introduced the Type 3 sulfides. The gold content across all three sulfide types is remarkably consistent, and overlaps completely between the three types of sulfides (average AuType1 = 0.41±0.25 ppm; AuType2 = 0.84±1.00 ppm; AuType3 = 0.69±0.58 ppm). This is within the range of peridotite hosted sulfides from south-eastern Australia, and south-eastern China (Fig. 3). The sulfide types are not limited to any one group, but the relative amount of each sulfide type does change, with Group III having more Type 3 sulfides, while the Groups I and II samples are dominated by Type 2 sulfides. The Group I samples are the only ones that contain any Type 1 sulfides.
Figure 1. Svalbard metasomatism increase La/Yb and Sr, while decreasing
Ti/Eu ratios – a typical signature of carbonatitic metasomatism.
Figure 2. Trace element characteristics of sulfides in Svalbard xenoliths.
The sulfide chemistry broadly reflects changes in the metasomatic history defined by the clinopyroxene. There is also an increase in the absolute abundance of sulfides with increasing metasomatism, with a corresponding decrease in the average Au content in the sulfides. This indicates the gold was scavenged locally rather than introduced from an external source. However, there is a lack of correlation between La/Yb in clinopyroxene and Au/Ir or Pd/Ir in sulfides. This is typical for other sample sets (e.g. south-eastern China, south-eastern Australia, and Kaapvaal Craton), indicating a global decoupling sulfide and silicate metasomatism (Fig. 3).
This project is part of CCFS Themes 2 and 3, Earth Evolution and Earth Today, and contributes to understanding Earth’s Fluid Fluxes.
Contacts: Ed Saunders, Norman Pearson, Sue O’Reilly, Bill Griffin
Funded by: ARC CoE CCFS Foundation Project 1 (TARDIS); APA, MQPGRF
Figure 3. Gold content in Svalbard sulfides compared to other xenolith suites. Metasomatism has little effect on the average Au concentration within mantle sulfides.
Many world-class magmatic nickel sulfide deposits formed close to Earth’s surface and there are probably few easily accessible deposits left to be discovered. The opening of new “exploration space” is essential to guarantee a steady metal supply in the future. One promising approach involves exploration for magmatic nickel-copper sulfide deposits that are hosted in the deep lithosphere, possibly at the crust-mantle boundary. However, very little is known about their genesis because such deep-seated deposits are rarely obducted to near-surface levels. The first step in developing exploration models for metal deposits in the deep Earth is to understand how metals are transported and concentrated at sub-crustal levels: an issue addressed by the CCFS project ‘Metal Sources and Transport Mechanisms in the Deep Lithosphere’.
This project includes two detailed case studies: the first is the Ivrea-Verbano Zone of Italy (Fig. 1) and the second is the Archaean Thrym Complex of southeastern Greenland: these are among the few known areas where magmatic nickel-copper sulfide deposits occur in rocks that came from the deep lithosphere. How many more are there? Nobody knows, because the current dominant ore-genesis models, are largely focused on terrains that contain rocks from the upper crust while neglecting areas with outcropping deep lithosphere.
Figure 1. Field work in the Ivrea-Verbano Zone of Northwest Italy.
The formation of magmatic nickel-copper sulfide deposits in rocks from the deep lithosphere appears to be directly linked to the occurrence of metasomatic fluids, which are most evident in the Ivrea-Verbano Zone as a series of peridotitic, pipe-like intrusions up to 300 metres in diameter. These pipes contain water-rich magmatic minerals, such as phlogopite and amphibole, which are closely associated with nickel, copper and iron sulfides (Fig. 2). These minerals have isotopic signatures indicative of a mantle source, so the Ivrea-Verbano Zone is an excellent natural laboratory to investigate the role of fluids in the transport and concentration of metals in the deep Earth.
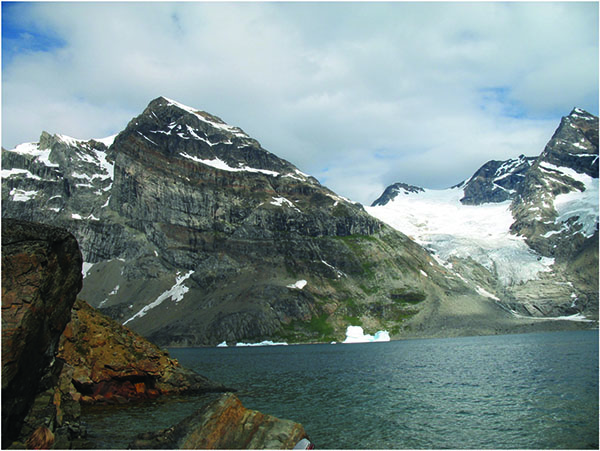
Trace-element data (LA-ICPMS) for amphibole and phlogopite from several pipes in the Ivrea-Verbano Zone suggest that juvenile, carbonate-rich hydrous fluids played an important role in the genesis of the pipes. The metasomatic fluid carried nickel, iron and copper and caused the parental rock to partially melt. The resulting melts evolved into the volatile-rich, pipe-like intrusions that reached sulfide-saturation during their emplacement, forming the sulfide deposits that we see today.
The Thrym Complex of southeastern Greenland is part of the North Atlantic Craton and is made up of migmatitic orthogneiss, narrow bands of mafic granulite, ultramafic and possible meta-sedimentary rocks, and alkaline-carbonatitic intrusive rocks. The narrow bands of mafic granulite are interpreted as mafic volcanic or gabbroic rocks from the lower crust. Ni-Cu-sulfide mineralisation can be found in the mafic granulite units and is most significant next to ultramafic bodies locally found within the mafic granulite bands. Sulfide mineralisation is also hosted in shear zones associated with the later part of the 2790-2700 Ma Skjoldungen Orogeny. The mafic and ultramafic rocks that host the mineralisation all show evidence for interaction with an incompatible-element-rich fluid at some point in their evolution.
This project is part of CCFS Theme 3, Earth Today and contributes to understanding Earth’s Fluid Fluxes.
Contacts: Marek Locmelis and Marco Fiorentini
Funded by: Foundation Project 2a: Experimental determination of metal sources and transport mechanisms in the deep lithosphere
Figure 2. Spectacular outcrops in Southeast Greenland.
Deformation in the mantle is the cause of brown and pink colours in diamonds: brown diamonds are worth relatively little, while pink ones can bring very high prices indeed. The colour of brown and pink diamonds commonly is confined within {111} lamellae created during deformation, and this spatial phenomenon is commonly referred to in the gem trade as graining. Despite this inherent relationship between plastic deformation and pink colour in diamonds, the exact internal crystal defect responsible for the colour absorption has not yet been identified.
Recent studies (CCFS publication #211) have identified the {111} deformation lamellae in natural pink and experimentally-deformed brown diamonds as micro-twins. However, we cannot assume that all brown and pink diamonds will contain micro-twins.
The Argyle Diamond Mine.
The Argyle diamond mine in Australia produces 90 to 95% of the world’s pink diamonds. However, a recent study has shown that pink diamonds from Argyle have characteristics very different from the even rarer pink diamonds from other localities (Gaillou et al., 2010; Diamond & Related Materials). We have investigated these two groups of pink diamonds to better understand their differences and the processes of deformation in diamonds deep within the Earth.
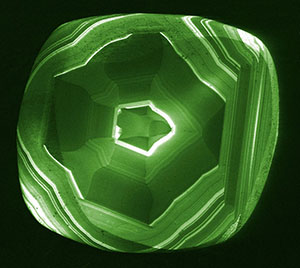
Scanning electron microscope (SEM)-based electron backscatter diffraction (EBSD) is an ideal tool for investigating the deformation of diamonds and identifying the presence of micro-twins. We have used this technique available in the CCFS Geochemical Analysis Unit at Macquarie to study a set of diamonds provided by the Diamond Trading Company. Combining EBSD with cathodoluminescence (CL) imaging, another SEM-based technique, we have confirmed that pink diamonds from Argyle mine have a very unusual growth pattern, while pink diamonds from other localities have previously described growth patterns, where the {111} deformation lamellae cut across the growth stratigraphy (Fig. 1). Our work has confirmed that these {111} deformation lamellae are micro-twins, and that these features are not observed in the pink diamonds from Argyle. Work is ongoing to fully understand the mechanism by which the micro-twins form and what they tell us about the deformation environment experienced by the diamonds and their subsequent history deep within the Earth.
CL image of sector-zoned diamond.
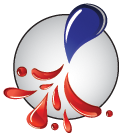
This project is part of CCFS Theme 2, Earth Evolution, and contributes to understanding Earth’s Fluid Fluxes.
Contacts: Dan Howell, Bill Griffin
Funded by: ARC CoE CCFS Foundation Project 8
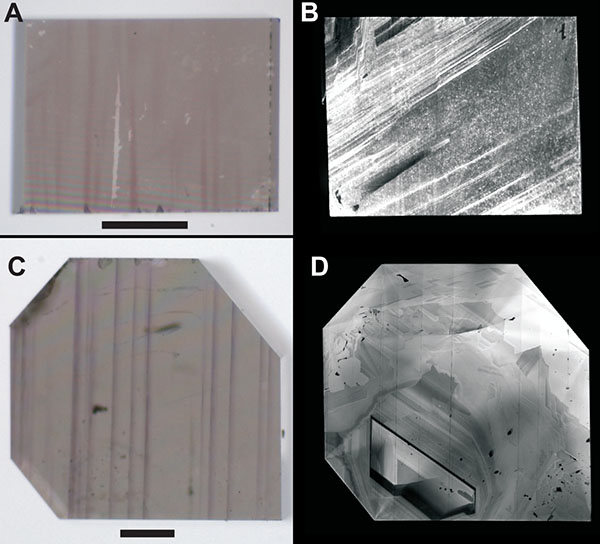
Figure 1. Images of two different pink diamonds. Images A & B are of an Arglye pink diamond, under plane light and CL respectively. The pink colour is less restricted to the {111} lamellae, and in the CL image the colour is very grained and does not correlate clearly with the {111} lamellae. Images C & D are from a pink diamond from South Africa. The plane light photo (C) shows the pink colour is very clearly retained in the {111} lamellae while the bulk of the diamond is colourless. These lamellae, shown to be micro-twins by EBSD analysis, are clearly picked out in the CL image (D), cross cutting the primary growth stratigraphy of the diamond. Scale bars represent 0.5mm.
Interpretation and prediction of past and future behaviour of polar and glacial ice is a major challenge, especially in view of changing climate. We performed unique deformation experiments to advance our knowledge of the effects of temperature changes and deformation rates on the flow properties of ice. In-depth knowledge of these properties is essential in order to develop reliable climate models incorporating the effects of polar and glacial ice mass flow and their reduction through time.
The team used a novel, world-first technique to investigate the deformation of ice. Heavy-water ice (D2O) was used as a direct analogue for natural-water ice (H2O). The use of heavy-water ice offers the unique opportunity to utilise neutron diffraction analysis in order to simultaneously monitor the flow properties, microstructure and orientation properties of ice. Laboratory grown polycrystalline samples were shortened up to 40% at variable temperatures and at three different constant deformation rates. Results show that ice exhibits a highly dynamic deformation behaviour. With deformation grain orientations change rapidly and distinctly. The processes that govern the flow properties of ice are changing with increasing temperature and amount of deformation. The competition of these processes is highly dynamic but predictable and this competition defines ice dynamics and needs to be incorporated in ice mass modelling.
Within this ongoing project the research team will expand the analysis to so called “dirty” ice, ice which is mixed with rock powder. The behaviour of “dirty” ice is not well understood, despite it’s growing importance as ice masses become more and more rock powder laden in responce to climate change.
This project is relevant to CCFS theme 3, Earth Today and contributes to understanding Earth’s Fluid Fluxes.
Contact: Sandra Piazolo
Funded by: ARC Furture Fellowship, Bragg Institute, ANSTO Lucas Heights
Figure 1. (left) Studying a rapidly flowing glacier which drains the Greenland ice cap, West Greenland. The rapid flow is a direct effect of elevated temperatures. Understanding the underlying principles of ice mass flow is essential for the prediction of climate change effects. (right) Experimental ice microstructures before deformation (top), after 10% deformation at low strain rates (middle) and high strain rates (bottom); optical micrographs where different colours signify different orientations, width of image is 4.8 mm.